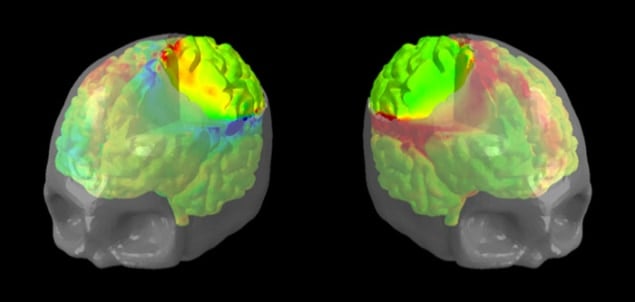
Simple models of soft solids can be used to accurately assess the damage that may be caused by an evasive brain operation known as a craniectomy. That’s the finding of a team of researchers from the UK and America who used the mathematics of their simple model to develop a realistic simulation of such procedures, which they hope will help to improve patient outcomes.
Traumas such as strokes, tumours and traumatic brain injury can cause the brain to swell. The resulting increase in intracranial pressure can inhibit blood flow and cause parts of the brain to die, with long-lasting or fatal consequences. To relieve the pressure, surgeons may decide, as a last resort, to remove a large section of the patient’s skull and leave a hole in the bone, a procedure known as a craniectomy. In modern medicine, craniectomies have been used for more than a century, but there is archaeological evidence to suggest that similar procedures were being performed thousands of years ago. The procedure is controversial, however, as failure rates are high and outcomes can be poor.
Under pressure
Earlier this month, researchers at the University of Cambridge published the results of a 10 year study on craniectomy. More than 400 people who had suffered traumatic brain injury and had high intracranial pressure were randomly assigned to receive craniectomy or medical care. Six months after the head injury, a quarter of the patients who received a craniectomy had died (27%), compared with half who received medical care (49%). But, the surviving craniectomy patients were more likely to be in a vegetative state or have a severe disability.
Damage occurs because the procedure causes the brain to deform as it bulges through the opening. For example, blood vessels and brain tissue can be squashed, leading to herniation and loss of blood flow, and stretching can damage or kill axons – the long part of nerve cells.
To improve outcomes, it is important to understand the stresses that develop in the brain after the operation. Alain Goriely, a mathematician at the University of Oxford, and colleagues at Stanford University in the US, the University of Oxford and the University of Exeter, decided to tackle this by looking at a simple physical problem: bulging in soft solids. Starting with mathematical models of simple geometries – a cylinder and a sphere – they looked at what happens when a constrained soft-solid that is swelling is only allowed to expand through a single circular hole. What shape does the bulge form? What stresses and stretches develop?
Stretch and slide
The simulations revealed three potential issues that could cause damage. Stretching in the centre of the bulge, compression at the edge of the opening, and regions of high shear stress around the opening, where outward sliding is constrained. The first two of these, in particular, correspond with known issues following craniectomy – axon stretch and constriction of blood flow and cells.
The researchers found that moderate swelling can produce harmful forces. Their estimates show that if the deformation is restricted so that all fibre strains remain below 20%, a relatively modest-sized bulge is produced compared to those seen following craniectomy. Yet, it is know that axons can be damaged by strains as low as 4%.
Goriely says that while most scientists believe stretch causes the most axon damage, the physics of materials shows that “shear is naturally associated with damage”. He adds: “We now believe that shearing is equally dangerous to the brain. Indeed, whereas tissues can resist compression and axons can recover from small extensions, shearing is associated with tissue rupture.”
The team also created a realistic model of a brain encased in a skull, based on magnetic resonance imaging of a female head. Simulations of a craniectomy, using the mathematics developed earlier, reproduced the same findings as seen in the simple models. “The beauty of the problem is that these three mechanisms appear to be universal,” says Goriely, “They hold for idealised geometries like a circular hole in a swelling sphere, but also for a real craniectomy opening in a human brain.” The model-brain simulations showed that a 10% expansion in brain tissue produces axon strain, compression and shear that are above the known damage thresholds for axons.
Difficult compromise
Goriely says that from a “physics point of view”, a craniectomy is a choice between high compressive stresses due to swelling or high strains due to large deformations. “Our method can help shed light on this difficult compromise, and suggest modifications of the procedure that induce less damage,” he adds. The researchers hope that once the computer modelling has been validated, it can help rationalize patient selection, and optimize opening location, shape and size to improve outcomes.
Angelos Kolias, a clinical lecturer in neurosurgery at the University of Cambridge, who was also a co-author of the 10 year study, told physicsworld.com that the craniectomy trial had “confirmed the life-saving nature of the operation”, but cautions that the quality of life of patients following this operation must be closely monitored.
“Bio-engineering approaches, such as the one described, are very promising as they can help us better understand the effects of craniectomy on the brain tissue,” adds Kolias. “Additionally, bio-engineering approaches could help us answer clinical questions regarding the optimal size and location of craniectomy in a patient-specific manner. We believe that a multidisciplinary approach to the issue of brain deformation following craniectomy will play a central role in the efforts to optimize patient outcomes.”
The research is described in two papers in Physical Review Letters and Computer Methods in Applied Mechanics and Engineering.