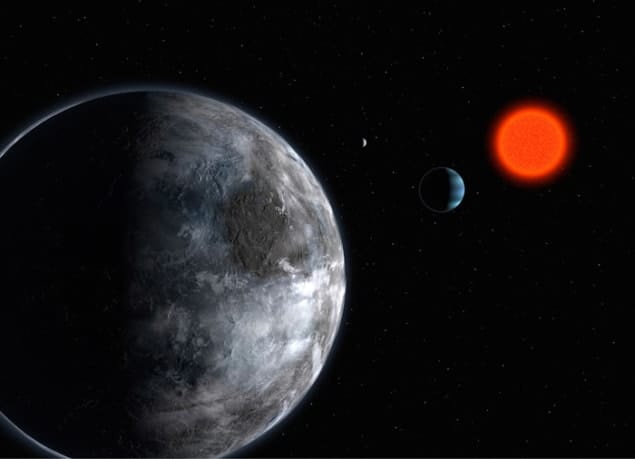
Rocky exoplanets with masses that are between 2–10 times that of the Earth may not have the long periods of volcanic activity that are thought to be essential for life to evolve. That is the conclusion of a new study by scientists in the US and Germany into the rate with which these “super Earths” cool. The research also suggests that understanding the behaviour of a super planet’s interior over long periods of time can provide important information about conditions on its surface.
Astronomers have so far found more than 600 super Earths orbiting stars other than the Sun – and many more are expected to be discovered by the Kepler space telescope and other future missions. Super Earths currently offer astronomers the best opportunity to look for signs of extra-terrestrial life because they are easier to find and study with today’s telescopes than are Earth-sized exoplanets.
If a super Earth were in the habitable zone of its star – where, in principle, the surface of the planet could be at the right temperature to have liquid water – such an exoplanet could sustain life. Being in the habitable zone alone is, however, not enough because the conditions on the surface of the super Earth must be conducive to life.
Volcanoes needed
The new study is by Vlada Stamenkovic of the Massachusetts Institute of Technology and colleagues from the DLR Institute of Planetary Research in Berlin. It suggests that super Earths are unlikely to have the long periods of volcanism that is believed to play an important role in making the surface of a planet suitable for the emergence of life. In the case of our own planet, heat transferred from deep within the Earth via convection in the mantle makes the continents shift round like large plates, with this “plate tectonics”. This triggers long periods of volcanic activity, which spews out so much CO2 that it leads to greenhouse warming. This keeps the Earth’s surface cosily warm, with most of the water being liquid rather than frozen.
Ongoing volcanism also plays an important role in the geological carbon cycle, whereby CO2 held in rocks is put into the atmosphere by volcanoes and then incorporated back into the planet’s interior via rock weathering and plate tectonics. This cycle is believed to contribute to Earth’s relatively stable climate, which in turn is believed to be a prerequisite for life.
Stamenkovic and colleagues have looked at a number of different factors that could affect the conditions on the surface of a super Earth. They began by considering the viscosity of the interior of a super Earth, which determines how quickly heat moves via convection from the hot centre of a planet to its much cooler surface. Planets with high-viscosity interiors transfer heat more slowly than those with low viscosity.
Pressure-dependent viscosity
Most calculations to date have used a formulation for viscosity that is dependent only on temperature but not pressure – a formulation that doesn’t even work for the Earth, according to Stamenkovic. Instead, the team argues that at the greater pressures found in super Earths the correct formulation for viscosity should also include a term that is a function of pressure. The result is a much larger viscosity than used in previous studies of super Earths.
The team then looked at how the temperatures within a super Earth would change over its lifetime – because both the temperature and pressure define the viscosity inside a planet. They first calculated what would happen if super Earths were as “cool” as generally predicted by previous studies. At these relatively low temperatures Stamenkovic and colleagues predict that there will be no convection in the lower mantle. In this “stagnant” scenario, the exoplanet cools slowly.
However, more recent studies suggest that super Earths are much hotter when they first form – perhaps even molten. Under this hot-formation scenario, the team found that convection will occur, albeit at a sluggish rate. In both cases the cooling of the mantle and the core would be inefficient, according to Stamenkovic.
Armed with this information, the team then looked at the implications of this sluggish cooling on the habitability of a super Earth. In both cases, the slow cooling of the core and mantle of a super Earth suggest that plate tectonics is less likely to occur. Stamenkovic points out, however, that the water content of the lithosphere also plays an important role in determining whether plate tectonics will occur, and might reverse such trends.
Laboratory experiments needed
According to Stamenkovic, this latest research shows the importance of understanding the time evolution of super Earths, rather than trying to calculate their steady-state properties. To gain a better understanding of how heat is transferred in super Earths, Stamenkovic says that more high-pressure experiments need to be done in labs here on Earth and that more data on the densities and atmospheres of super Earths is required. To gather this information, he supports a number of low-cost space telescopes that would each focus on one nearby super Earth for the long periods of time needed to gather enough data. Such a project has been proposed by a team at MIT led by Sara Seager and is called ExoplanetSat.