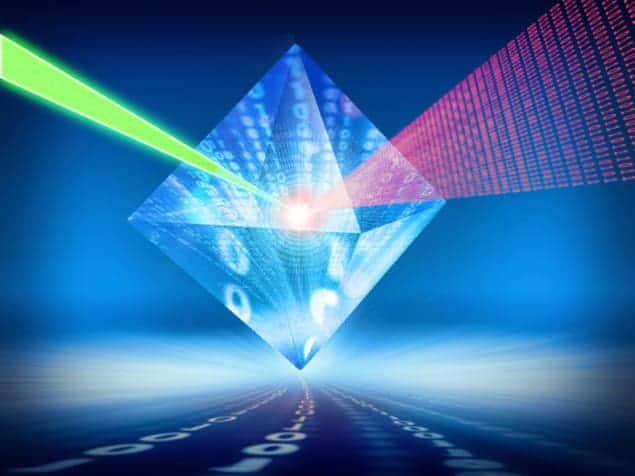
Two independent teams of physicists have created quantum memories, based on nuclear spin, that push the limits of how long quantum information can be stored in solid-state devices. One device – based on a doped crystal of ultrapure silicon-28 – is able to store data for more than 3 min. The other memory – based on nitrogen vacancies (NVs) in an isotopically pure carbon-12 diamond crystal – achieved a storage time of 1.4 s. While this latter result might not seem as impressive, the diamond memory works at room temperature, whereas the silicon device must be cooled to a temperature of below 2 K.
The new findings are of interest to anyone wishing to build quantum computers, which can, in principle, use quantum mechanics to perform certain calculations much faster than conventional computers. In particular, the results could help to tackle a fundamental problem for quantum-computing researchers that concerns how quantum information (qubits) are stored and manipulated.
The problem is that for a qubit to maintain its quantum nature, it must be isolated from its surroundings to stop it being destroyed in a process called decoherence. But to manipulate quantum information, a qubit must be controlled externally – and this connection to the outside world will lead to decoherence. One solution is to first store the quantum information in a very isolated qubit, before transferring it to a more accessible qubit where it can be quickly processed, then returning it to the safety of isolation.
Although ions trapped in a vacuum have been used to create memories that can store qubits for tens of minutes, many physicists believe that any practical quantum-computing device must be made from solid-state materials that can be integrated with conventional electronics. As a result, some researchers have pinned their hopes on what is perhaps the most isolated system in a solid – a nuclear spin. If an external magnetic field is applied to such a nuclear spin, quantum information can be stored in the spin state of the nucleus – whether it is pointing up or down with respect to the magnetic field, for example.
No nuclear spins in the background
The new silicon memory was made by Mike Thewalt and colleagues at Simon Fraser University in Vancouver, Canada, and Oxford University, UK. They worked alongside physicists at the PTB standards lab, the Leibniz Institute for Crystallography and Vitcon Projectconsult – all in Germany – to create a memory based on the nuclear spin of a phosphorous-31 nucleus embedded in silicon.
The diamond memory, meanwhile, was created at Harvard University in the US by Mikhail Lukin and his team, with help from physicists at the California Institute of Technology in the US and the Max Planck Institute for Quantum Optics in Garching, Germany. Their device uses a carbon-13 nuclear spin near a NV in diamond supplied by Luxembourg-based firm Element Six. Silicon-28 and carbon-12 were chosen because both nuclei have zero nuclear spin and therefore will not interact with the phosphorus-31 and carbon-13 nuclear spins, respectively – a process that leads to decoherence.
Both schemes use electron spin as a qubit that can be manipulated externally to exchange quantum information with memories based on nuclear spin. In the silicon memory, the electron spin is associated with the phosphorus atom itself, whereas the diamond memory uses an electron from the NV.
In both cases the connection between electron and nuclear spin comes via the “hyperfine interaction” between the spin magnetic moments of the electron and nucleus. The result is a tiny splitting of electron energy levels, which affects how the material absorbs and emits light. This allows the transfer of quantum information to be controlled using a sequence of laser and microwave pulses. By using carefully chosen sequences of laser and microwave pulses, both teams were able to transfer the quantum states of their electron-spin qubits to their nuclear-spin memories. The quantum data are then retrieved using further sequences of laser and microwave pulses.
Rogue interactions
An important challenge facing both teams was the presence of isotopic impurities of silicon-29 or carbon-13 in their devices. These devices have magnetic moments that will couple with both the electron and nuclear spins of interest, thereby upsetting energy levels and causing decoherence. To deal with this problem, both groups use schemes that place their systems into states in which these rogue interactions are minimized.
The diamond team go one step further and actually “switch off” the hyperfine interaction between qubit and memory while the datum is stored, which allows them to boost the storage time to more than 1 s.
Thewalt told physicsworld.com that the team is looking at using bismuth nuclei in silicon-28. As well as having a more pronounced hyperfine splitting, bismuth has a nuclear spin of 9/2, which gives the system a much richer hyperfine-state spectrum. “We are also interested in the nuclear spin of the ionized phosphorus donor in these same samples, which might have an even longer coherence time, and retain this long coherence time to higher temperatures,” he adds.
As for Lukin, he believes that his team’s memory could soon find applications beyond quantum computing, saying that the device’s sensitivity to magnetic fields “may enable a range of novel and disruptive quantum-sensor technologies, such as those being targeted to image magnetic fields on the nanoscale for use in imaging chemical and biological processes”.
The findings are reported in two papers in Science.