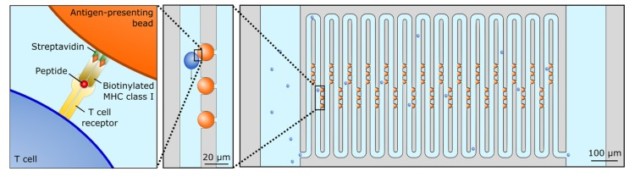
T-cells, which recognize pathogens such as viruses and bacteria, play a crucial role in the immune system. The affinity (or stickiness) with which T-cells bind to pathogen-based biomolecules determines how effective they will be in mounting an immune response.
Some emerging cancer treatments employ T-cells trained to recognize cancer cells and, for such therapies, the ability to sort T-cells based on affinity could potentially improve clinical outcomes. Unfortunately, existing techniques for testing affinity are low-throughput and not suitable for clinical use. Now, a team of researchers at Massachusetts Institute of Technologyhas used microfluidic technology to take the first step towards high-throughput affinity-based T-cell sorting (Biomicrofluidics11 064103).
As T-cells move throughout the body, they scan for foreign invaders using a biomolecular sensor called the T-cell receptor (TCR). The T-cell has TCRs on its surface, and as the T-cell crawls, these TCRs stick to pMHCs, signalling molecules that can indicate the presence of pathogens. Some TCR-pMHC bonds are stronger than others, and if the two bind with sufficient affinity then the T-cell will initiate an immune response.
To test the affinity of an individual TCR-pMHC pair, the T-cell physically pulls on the TCR to try to break the two apart. The longer the TCR-pMHC pair can stay bonded under tension, the more likely the T-cell is to initiate an immune response. Single-molecule testing techniques can mimic this mechanical test by applying tension to a single TCR-pMHC bond, but such approaches can only test one cell every few minutes. That is far too slow to sort through the millions of cells needed for T-cell-based therapies. As such, the team, led by Scott Manalis, is exploring new approaches for increasing throughput by using microfluidics.
Microfluidics uses micrometre-scale fluid-flow channels for applications where very small volumes of liquid must be moved around (for example, small-volume chemistry or single-cell manipulation). The technology is often used in conjunction with a microscope to sort cells based on visible cues such as size, shape and colour. In this study, the team used a clever trick to estimate the strength of TCR-pMHC interactions in single cells flowing through a microfluidic device.
Manalis and colleagues designed a long, zig-zagging “serpentine” microfluidic channel through which they flow individual T-cells. They also embedded pMHC-coated beads in the channel. As the T-cells move through the channel, they sometimes brush up against the pMHC-coated beads. The T-cells’ TCRs then interact with the pMHC on the beads.
Importantly, the force of fluid flow is transmitted to the TCR-pMHC bonds, mimicking the mechanical test used naturally by T-cells and ultimately leading to bond rupture. The T-cells continue to flow through the channel, but their flow velocity decreases with increased affinity of TCR-pMHC interactions. The researchers measured this reduction in flow velocity using a standard microscope, observing a significant reduction compared with T-cells flowing past non-pMHC-coated beads.
By using a microfluidic device to mimic the mechanical biomolecular test used by T-cells, the team observed a modest increase in testing throughput, to 4-5 cells per minute. “Our future efforts will be geared towards further increasing throughput,” says first-author Max Stockslager. He noted that unlike previous techniques, this sorting method is scalable as several microfluidic channels can be imaged at once. Looking forward, the researchers also hope to translate this technique into a clinical tool for improving T-cell-based therapies.