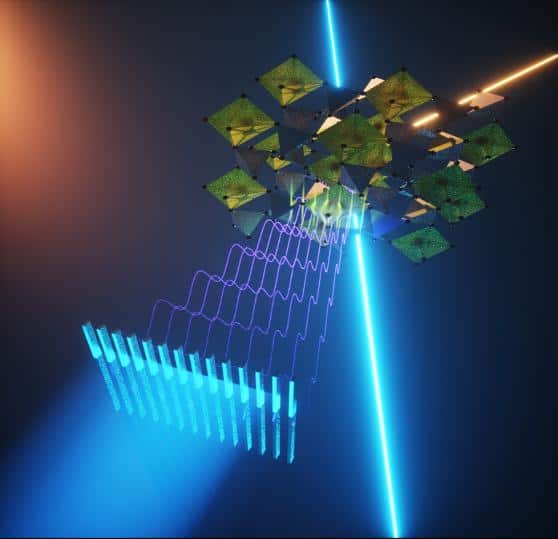
Researchers in Switzerland have made a popular materials characterization technique accessible to ultrafast X-ray laser light for the first time. The new approach allowed the team to film atomic processes that play out in mere femtoseconds, and it could make the technique – known as transient grating spectroscopy – an important complement to other methods such as inelastic neutron and X-ray scattering for imaging materials at atomic-scale resolution.
Transient grating spectroscopy uses two interfering laser beams instead of just a single beam to characterize materials. In this non-destructive technique, researchers use these beams to create a temporary interference pattern (the transient grating) that repeats at regular intervals, known as Talbot planes. The characteristics of the sample can be probed by placing it in one of these planes.
The distance between the stripes in the interference pattern depends on the wavelength of light pulses used to create the pattern. For wavelengths spanning the visible to the ultraviolet part of the electromagnetic spectrum, this distance is on the order of hundreds of nanometres – meaning that if the sample being imaged contains any features smaller than this, they will not be visible. Using radiation with shorter wavelengths, such as X-rays, can improve the technique’s resolution. However, crossing and positioning two X-ray beams well enough to generate a grating with nanometre stripe size has proved challenging.
Very hard-X-rays from the SwissFEL
Together with colleagues in the US and Italy, researchers led by Cristian Svetina of the Paul Scherer Institute (PSI) and Jérémy Rouxel of the EPFL overcame these difficulties to make a transient grating from very hard-X-ray beams. The radiation they used was produced at the SwissFEL facility and has an energy of 7.1 keV, which corresponds to a wavelength of 0.17 nm – the diameter of a medium-sized atom. This technical feat allowed the researchers to characterize a sample of bismuth germanate with a resolution down to individual atoms, at ultrashort exposure times of fractions of femtoseconds (10-15 s).
Talbot planes
The new approach involves first sending ultrashort X-ray pulses onto a transmission phase grating, or mask. This comb-like structure is made of diamond, which is robust to high-energy X-rays, and was specially fabricated by PSI team member Christian David. The “teeth” of the comb are spaced 2 microns apart and break up the X-ray beam into finer, partial, beams that interfere behind the diamond grating to create the transient grating diffraction pattern. This pattern is a faithful image of the diamond grating and is repeated at each Talbot plane.
“If we place a sample in one of these Talbot planes, some atoms within the sample become excited – just as if the sample were sitting at the location of the diamond grating itself,” Svetina explains. Crucially, only the atoms exposed to the X-rays in this periodic modulation are excited, and Svetina notes that this is the technique’s main attraction, as it allows the team to selectively excite areas of interest in a sample.
No unwanted background signals
Another big advantage of the method is that does not produce any unwanted background signals. “If the atoms are excited, you see a signal; if they are not excited, you see nothing,” Svetina explains. This is extremely valuable when measuring samples that emit only weak signals.

World’s thinnest diffraction gratings made from graphene
Members of the team note that characterizing materials on the atomic scale is becoming important for microchip manufacturers as they seek to study and improve nano-sized features on their chips. Studies like this one, which appears in Nature Photonics, will also make it possible to glean fresh information about various quantum phenomena, including heat transport in semiconducting materials and the processes involved when individual computer bits are magnetized.