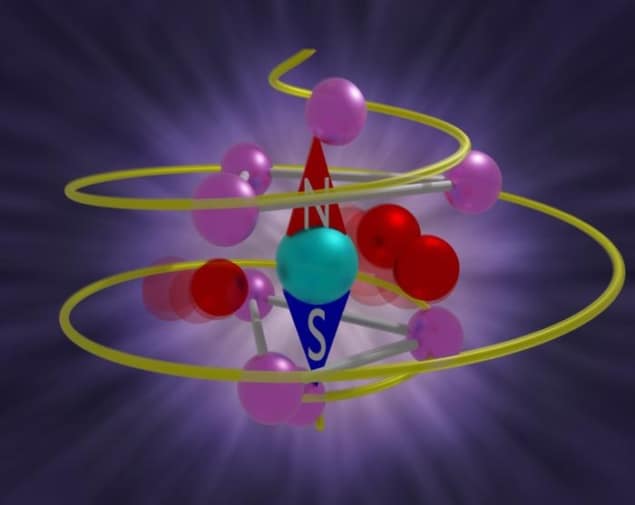
When a material’s atomic lattice vibrates, it produces quasiparticles known as phonons, or quantized sound waves. In certain materials, vibrating the lattice in a corkscrew pattern will make these phonons chiral, meaning they take on the “handedness” of the vibration that produced them. Now, researchers at Rice University in the US have found that these chiral phonons have a further effect: they can make the material magnetic. This finding could be used to induce properties that are difficult to find in naturally-occurring materials.
One such hard-to-find property concerns violations of electrons’ time-reversal symmetry. In essence, time-reversal symmetry implies that electrons should behave the same regardless of whether they are moving forwards or backwards in a material. The most common way of violating this symmetry is to place the material in a magnetic field, but for some possible applications, this is not practical.
Previously, the thinking was that atoms move too little and too slowly in their crystal lattice to affect electrons’ time-reversal symmetry. In the new work, however, a Rice team led by Hanyu Zhu found that when atoms rotate around their average positions in the lattice at a rate of about 10 trillion revolutions per second, the resulting spiral-shaped vibrations – chiral phonons – break the electrons’ time-reversal symmetry and give them a preferred time direction.
“Each electron possesses a magnetic spin that acts like a tiny compass needle embedded in the material, reacting to the local magnetic field,” explains team member Boris Yakobson. “Chirality – also called handedness because of the way in which left and right hands mirror each other without being superimposable – should not affect the energies of the electrons’ spin. But in this instance, the chiral movement of the atomic lattice polarizes the spins inside the material as if a large magnetic field were applied.”
The magnitude of this effective magnetic field is about 1 Tesla, Zhu adds, making it comparable to that produced by the strongest permanent magnets.
Driving the motion of a lattice of atoms
The researchers used a rotating electric field to drive the motion of a lattice of atoms in a spiral pattern. They did this in a material called cerium fluoride, a rare earth trihalide that is naturally paramagnetic, meaning the spins of its electrons are normally randomly oriented. They then monitored the electronic spin in the material using a short light pulse as a probe, firing the light at the sample with varying time delays after applying the electric field. The polarization of the probe light changes according to the spin direction.
“We found that when the electric field had gone, the atoms continued to rotate and the electronic spin kept flipping to align with the rotational direction of the atoms,” explains Zhu. “Using the flipping rate of the electrons, we can calculate the effective magnetic field they experience as a function of time.”
The calculated field agrees with that expected from the team’s models of driven atomic motion and spin-phonon coupling, Zhu tells Physics World. This coupling is important in applications such as writing data on hard disks.

Researchers find ‘lost’ angular momentum
As well as shedding new light on spin-phonon coupling, which is still not fully understood in rare earth halides, the findings could enable scientists to develop materials that can be engineered by other external fields such as light or quantum fluctuations, Zhu says. “I have been thinking about this possibility since my post-doc at UC Berkeley, when we performed the first time-resolved experiments to verify the rotation of atoms in two-dimensional materials,” he explains. “Such rotational chiral phonon modes were predicted a few years back and since then I kept wondering: could the chiral motion be used to control electronic materials?”
For now, Zhu stresses that the work’s main applications lie in fundamental research. However, he adds that “in the long run, with the help of theoretical studies, we may be able to use atomic rotation as a ‘tuning knob’ to enhance properties breaking time-reversal and seldom found in natural materials, like topological superconductivity”.
The Rice researchers, who detail their present work in Science, now hope to apply their method to explore other materials and look for properties beyond magnetization.