
“Properly connected, proteins are the world’s best molecular wires,” says Stuart Lindsay, Director and Professor at the Biodesign Center for Single Molecule Biophysics at Arizona State University (ASU). His comments refer to recent experiments at ASU to measure the conductance of single proteins between electrodes for the first time with what he describes as “staggering” results that may have uses for direct, label-free, sensitive, and very selective (background-free) single-molecule detection as well as protein motion sensing. “Measurements on peptides (small protein chains) show they are the world’s worst molecular wires,” he adds. So what changed?
Contact control
The conclusion that proteins have a terrible conductance tallies well with their general physical characteristics – they lack both electronic conduction bands and high levels of structural order. Previous experiments have attempted to investigate the conductance of proteins by injecting electrons from optically excited chromophores, but contacting the molecule to an electrode allows studies of the response to injection of electrons with much lower energies. However so far these have struggled against the uncertainty in the number of molecules contacted, the size of the gap, the nature of the contacts, and possible ionic contributions to the current measured.
Here scanning tunnelling measurements present significant advantages over macroscopic contact devices as Lindsay explains because they allow for single-molecule contacts. “In addition, we carry out the experiments under electrochemical potential control so we can be certain there are no ionic currents and we use chemically well-defined contacts, so that we know (and can control) the metal-molecule interface.”
The resulting conductances measured in the nanosiemens range over distances of several nanometres. Having ruled out ionic currents the researchers were also able to eliminate possible meaningful contributions from tunnelling, which would be five orders of magnitude weaker. Crucially, while the conductance varied little with the length of the protein, the conductance was highly dependent on the chemistry of the electrodes, which the researchers functionalized with specific ligands for different proteins.
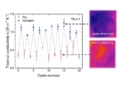
Squid-inspired proteins make new thermal switch
Current pathways
The researchers found that the chemistry of the contacts was so significant that weaker coupling to the hydrophobic interior of the protein provided a stronger current than a stronger coupling to the hydrophilic exterior. The researchers describe the role of contact chemistry in terms of the path the current takes either across the surface or through the interior. “We hypothesize that the hydrophobic interior of proteins is a wonderful place for electrons to propagate and that electrons are injected into the interior of the protein using specific ligands,” Lindsay tells Physics World.
The experiments also reveal fluctuations that set in above a threshold applied bias of 100 mV in magnitude. Comparison of the lifetime of the on states with the peak current magnitude indicate a single barrier that determines both the current and bonding strength at the contact. The magnitude of this barrier within the relevant exponential expression matches that of a hydrogen bond suggesting that a hydrogen bond may be playing the role of “weak link in the circuit”. The conductance values measured are also compatible with those calculated for thermally activated hopping over a 0.22–0.47-V barrier, the size of the hydrogen bond. “We have devised and implemented a new scheme to extract the electronic decay length, and temperature dependent measurements are underway as well,” adds Lindsay.
Significant findings
Further experiments demonstrated the potential for using the strong ligand specific conductance for single-molecule, highly specific, label- and background free electronic detection of IgG antibodies to HIV and Ebola viruses, and the researchers are now working on technological applications of the results. “The wonderful thing about sorting out the connections issue is that we have a “tool kit” for bioelectronics mapped out,” says Lindsay as he lists them. “(1) For wires, use ligand connected proteins. This not only makes a fantastic contact, but it also makes a self-assembling, directed contact. (2) Multivalent proteins complete circuits and can generate branched wiring. (3) Since the conductivity “reads” the internal state of the protein, enzymes can be wired as single molecule sensors with an exquisite response. Future results from the lab will illustrate this.”
What may take a little longer to unravel is the biological role of protein conductances. Here Lindsay points towards theoretical calculations by Gabor Vattay , which show that the calculated energy level distribution in a number of proteins matches that expected for a very unusual state of matter called “quantum critical”, which also matches certain previous observations of giant protein conductance fluctuations. “If this is true, then proteins must have evolved a rather special structure. So why?” asks Lindsay. “I don’t know, but it is very interesting that ligand mediated contacts allow for sharing of electrons. Does this play a role in recognition?” he adds, highlighting the weaker contacts forged by strong, covalent modifications of residues on the outside of a protein compared with weaker contacts made by ligands that reach into the protein. In terms of biological significance, it seems these latest results raise at least as many questions as they answer.
Full details are reported in the Proceedings of the National Association of Science.