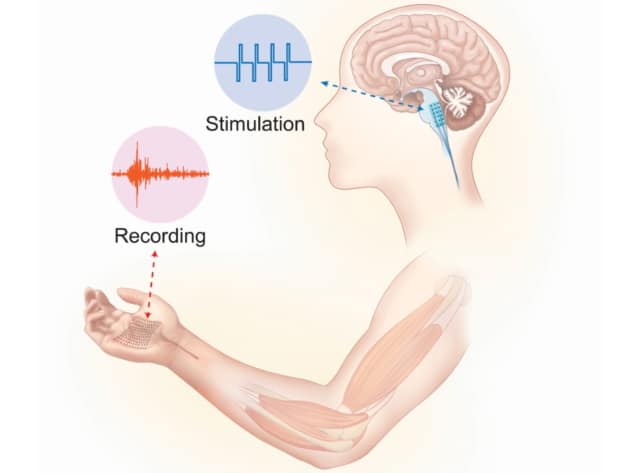
Wearable and implantable healthcare technologies can enable remote, multimodal monitoring for disease diagnosis and tracking, provide programmable, ongoing therapies for heart or retinal diseases, and even restore lost sensation via prosthetics. Yet, these technologies are limited by their material make-up, as they use rigid electrode materials that have limited mechanical compatibility with soft tissues and must be patterned to induce stretchability, limiting device density.
Conducting polymers are intrinsically soft, overcoming problems of tissue compatibility. They also benefit from a large volumetric capacitance that ensures high-quality signal recording and enhanced charge injection for stimulation. Despite these advantages, conducting polymers are not intrinsically stretchable, and existing chemical modifications used to induce stretchability result in conductivities that are too low for relevant bioelectronic applications.
That is why researchers at Stanford University, led by Zhenan Bao, used rational design to build a highly conductive, stretchable and photo-patternable material for use in bioelectronics, publishing their results in Science.
“We are the first to apply this supramolecular structure design into the field of stretchable conducting polymers,” explains Yuanwen Jiang, first author of the study.
Molecular engineering to meet complex design requirements
PEDOT:PSS – or poly(3,4-ethylenedioxythiophene):polystyrene sulfonate – is one of the highest performing and most investigated conducting polymer for use in bioelectronic devices. Previous attempts to modify the mechanical properties of PEDOT:PSS relied on blending approaches with ionic or molecular additives. But because they are not cross-linked to the conducting polymer, the additives are typically washed away in wet, biological environments, leading to large drops in conductivity and device performance.
“It a grand challenge for a single conducting polymer system to simultaneously possess high mechanical stretchability and electrical conductivity at the same time, especially when we micro-fabricate them down to cellular-level feature sizes for real device level applications,” says Jiang.

To meet this challenge, Jiang and colleagues had to design a supramolecular polymer additive for PEDOT:PSS molecule-by-molecule. Hypothesizing that high stretchability required mobile junctions with a large number of possible arrangements, they designed a polyrotaxane (PR) structure with cyclodextrin rings that can “slide” freely along the polymer backbone. This is the first work to introduce topology, or spatial arrangement, into the design of conducting polymers.
Having achieved stretchability, the researchers designed the polymer chemistry to meet all the requirements of integration with PEDOT:PSS. To enable water solubility and enhance interaction with the conducting polymer, they used polyethylene glycol as the backbone and the side chain. To introduce photo-patternability, they added methacrylates onto the side chains, allowing 2-µm feature sizes. Finally, they note that this multifunctional material could survive sulphuric acid treatment, which enhanced chain packing and thus overall conductivity.
This new material, which the team calls TopoE-S, outperforms all previous PEDOT:PSS modifications, achieving stable conductivity of 6000 S/cm, even at strains of up to 100%.
Outstretching the competition
The design of the bioelectronic interface is essential for applications in the body. “We want the best possible contact on interface, so that we can have the highest quality or signal-to-noise ratio in challenging dynamic environments,” said Jiang. The team has achieved that with the TopoE-S material.
When compared with microcracked gold, one the best stretchable electrodes, TopoE-S had a lower electrochemical impedance in all frequency ranges. This is because of the much larger electrochemical contact area of TopoE-S, which significantly reduces the interfacial impedance. All of these properties are essential for low-power electrical stimulation, the researchers note.
The TopoE-S array can be patterned into electrodes 100 µm in size, while its soft and elastic properties allow for conformal attachment to the skin. Thus, the team was able to fabricate devices to perform high-spatial-resolution electromyography (measurement of muscle’s electrical activity).
To demonstrate the capabilities of this advanced material, the researchers turned to octopuses, which provide a perfect test platform given the large deformation of their muscles. They found that upon electrical stimulation of the octopus arm, the electromyography device recorded muscle activity dynamics with consistently good signal-to-noise ratio, which was impossible with more rigid probes.
New medical therapy applications
Looking to the future, Jiang and colleagues are thinking about new tools that could improve clinical practice. “Through communication and collaboration [with doctors], we understand that there are unmet clinical needs. We try to design tools that can help improve care delivery,” he explains.
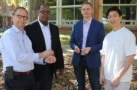
Stretchable organic photodiode could improve the performance of wearable devices
The TopoE-S material is designed to solve such unmet clinical needs. One example is the ability to map the brainstem prior to neurosurgery, to determine safe entry points for operation. Currently, this procedure is still performed using bulk electrodes that clinicians must manually move across the surface, greatly extending the time of the procedure.
The researchers patterned the TopoE-S into a high-density array and placed it on the brainstem of a rat, to allow location mapping with extremely high precision. By delivering current pulses to individual electrodes, they could control specific organ functions with little leakage current. Once again, their material outperformed rigid plastic probes that quickly caused severe damage to the fragile brainstem.