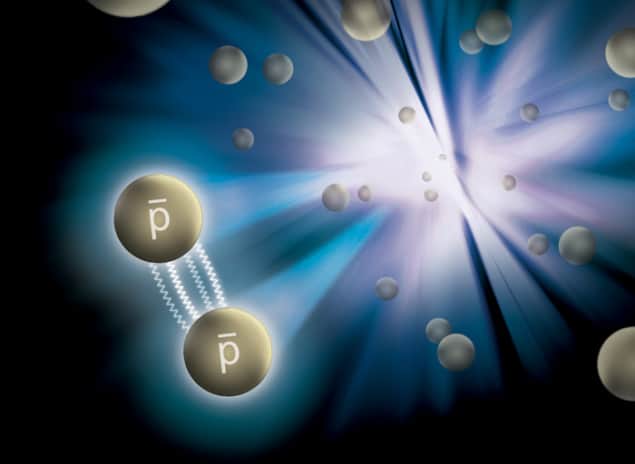
By examining the aftermath of the collisions of gold ions at close to the speed of light, an international team of physicists has measured the strong interaction between pairs of antiprotons for the first time. The researchers found that at very short distances, antiprotons – the antiparticles of protons – attract each other just as protons do. While this result was expected, it should improve our understanding of how antinuclei are held together. It also further strengthens the idea that charge, parity and time-reversal (CPT) symmetry is a fundamental symmetry of nature.
The strong interaction binds protons and neutrons together in atomic nuclei and it is also responsible for gluing together the quarks that make up protons, neutrons and other baryons. At very short distances, the strong force is attractive and much stronger than the electromagnetic force, which tends to push protons apart.
This latest experiment was carried out by the STAR collaboration at the Brookhaven National Laboratory’s Relativistic Heavy Ion Collider (RHIC). As well as showing that the force between antiprotons is attractive, the team was also able to measure two other important parameters – the scattering length and the effective range of the interaction.
It’s the mirror we think nature obeys
Daniel Kaplan, Illinois Institute of Technology
Brookhaven’s Aihong Tang explains that the team expected the interaction to be CPT symmetric, which means that antiprotons behave as protons do. CPT symmetry means that if you switched the charge and parity of a particle and reversed time, all of the laws of physics would remain the same. “It’s the mirror we think nature obeys,” says Daniel Kaplan of the Illinois Institute of Technology in the US, who was not involved in the work. No experiments, to date, violate CPT symmetry, and physicists currently accept it as “an article of faith”, he adds.
It is not surprising that the researchers did not find CPT violations, Kaplan says, because the conventional wisdom states that if such violations existed, they should be much weaker than the strong force. This means that STAR will have to make much more precise measurements if it hopes to reveal violations. “It will be some super-small interaction, so weak that we haven’t noticed it before,” Kaplan says. “The strong force is the strongest force there is. If you imagine some new mechanism that causes a CPT violation that no previous experiments have ever seen, how can it be big enough to be a significant part of the strong force?”
Lamppost search
While the results are not groundbreaking, it is useful to have another experimental confirmation of CPT symmetry, Kaplan explains. He describes the hunt for CPT violations this way: “Suppose you lost your keys at night. Where would you look? A normal person would say ‘I’d look where I think I dropped them.’ But a scientist would say ‘Let’s look under the lamppost because there’s actually light there.’.” The STAR collaboration looked for CPT violations where it had at least a chance of seeing them.
Performing the measurement is a numbers game, Tang says. The teams derived the result by observing the signature of the antiproton–antiproton interaction in 500 million gold–ion collisions. The team also made a similar measurement of the proton–proton interaction. Antiprotons are difficult to produce, and because they annihilate with regular protons to produce photons, they do not hang around, either. Each collision at RHIC produces thousands of particles but only 3–4% of these are antiprotons.
Useful parameter
This latest result will also help physicists to understand how antinuclei are bound together. Such experimental measurements are useful in quantum chromodynamics (QCD), the theory that describes the strong interaction. It is difficult to use QCD to calculate the strong force purely from theory, and this measurement of the antiproton interaction can be used as a parameter in the calculation.
In addition, by studying how antinuclei are bound together, physicists could learn how to make heavier antimatter nuclei. Several types of antinuclei have already been produced. These include antitritium – which is one antiproton bound with two antineutrons, and antihelium-4, which is two antiprotons bound with two antineutrons. However, Tang says that physicists are still a long way from producing antimatter for any sort of practical use.
The research is described in Nature.