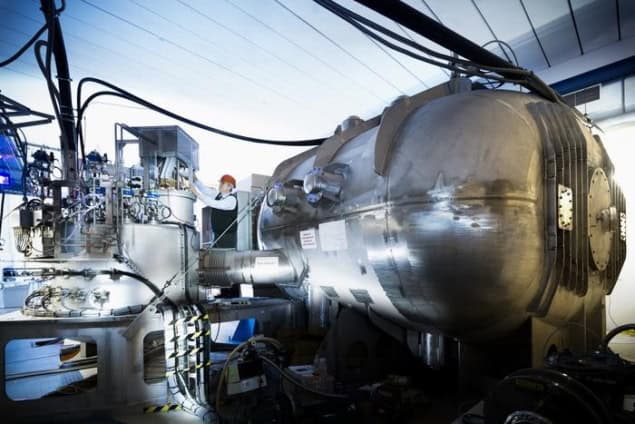
Subtle quantum effects within atomic nuclei can dramatically affect how some nuclei break apart. By studying 100 isotopes with masses below that of lead, an international team of physicists uncovered a previously unknown region in the nuclear landscape where fragments of fission split in an unexpected way. This is driven not by the usual forces, but by shell effects rooted in quantum mechanics.
“When a nucleus splits apart into two fragments, the mass and charge distribution of these fission fragments exhibits the signature of the underlying nuclear structure effect in the fission process,” explains Pierre Morfouace of Université Paris-Saclay, who led the study. “In the exotic region of the nuclear chart that we studied, where nuclei do not have many neutrons, a symmetric split was previously expected. However, the asymmetric fission means that a new quantum effect is at stake.”
This unexpected discovery not only sheds light on the fine details of how nuclei break apart but also has far-reaching implications. These range from the development of safer nuclear energy to understanding how heavy elements are created during cataclysmic astrophysical events like stellar explosions.
Quantum puzzle
Fission is the process by which a heavy atomic nucleus splits into smaller fragments. It is governed by a complex interplay of forces. The strong nuclear force, which binds protons and neutrons together, competes with the electromagnetic repulsion between positively charged protons. The result is that certain nuclei are unstable and typically leads to a symmetric fission.
But there’s another, subtler phenomenon at play: quantum shell effects. These arise because protons and neutrons inside the nucleus tend to arrange themselves into discrete energy levels or “shells,” much like electrons do in atoms.
“Quantum shell effects [in atomic electrons] play a major role in chemistry, where they are responsible for the properties of noble gases,” says Cedric Simenel of the Australian National University, who was not involved in the study. “In nuclear physics, they provide extra stability to spherical nuclei with so-called ‘magic’ numbers of protons or neutrons. Such shell effects drive heavy nuclei to often fission asymmetrically.”
In the case of very heavy nuclei, such as uranium or plutonium, this asymmetry is well documented. But in lighter, neutron-deficient nuclei – those with fewer neutrons than their stable counterparts – researchers had long expected symmetric fission, where the nucleus breaks into two roughly equal parts. This new study challenges that view.
New fission landscape
To investigate fission in this less-explored part of the nuclear chart, scientists from the R3B-SOFIA collaboration carried out experiments at the GSI Helmholtz Centre for Heavy Ion Research in Darmstadt, Germany. They focused on nuclei ranging from iridium to thorium, many of which had never been studied before. The nuclei were fired at high energies into a lead target to induce fission.
The fragments produced in each fission event were carefully analysed using a suite of high-resolution detectors. A double ionization chamber captured the number of protons in each product, while a superconducting magnet and time-of-flight detectors tracked their momentum, enabling a detailed reconstruction of how the split occurred.
Using this method, the researchers found that the lightest fission fragments were frequently formed with 36 protons, which is the atomic number of krypton. This pattern suggests the presence of a stabilizing shell effect at that specific proton number.
“Our data reveal the stabilizing effect of proton shells at Z=36,” explains Morfouace. “This marks the identification of a new ‘island’ of asymmetric fission, one driven by the light fragment, unlike the well-known behaviour in heavier actinides. It expands our understanding of how nuclear structure influences fission outcomes.”
Future prospects
“Experimentally, what makes this work unique is that they provide the distribution of protons in the fragments, while earlier measurements in sub-lead nuclei were essentially focused on the total number of nucleons,” comments Simenel.
Since quantum shell effects are tied to specific numbers of protons or neutrons, not just the overall mass, these new measurements offer direct evidence of how proton shell structure shapes the outcome of fission in lighter nuclei. This makes the results particularly valuable for testing and refining theoretical models of fission dynamics.
“This work will undoubtedly lead to further experimental studies, in particular with more exotic light nuclei,” Simenel adds. “However, to me, the ball is now in the camp of theorists who need to improve their modelling of nuclear fission to achieve the predictive power required to study the role of fission in regions of the nuclear chart not accessible experimentally, as in nuclei formed in the astrophysical processes.”
The research is described in Nature.