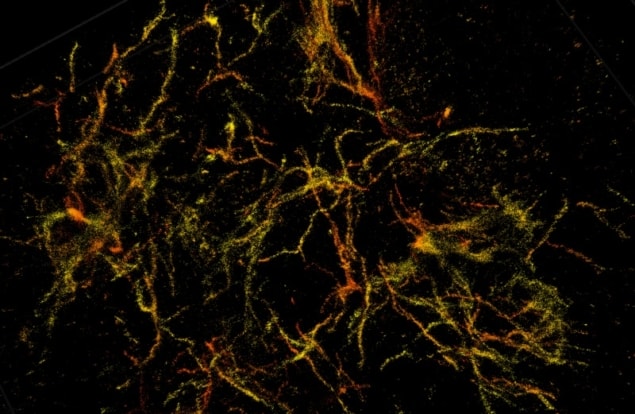
Alzheimer’s disease begins to develop 10 to 20 years before memory problems manifest, but currently, we are not able to clearly see why the disease starts. The earliest detectable evidence of pathological change leading to Alzheimer’s disease is the accumulation of waxy deposits called amyloid plaques in the brain.
Researchers at Purdue University have developed a super-resolution nanoscope that provides a 3D view of brain molecules with 10 times greater detail than conventional microscopes. Indiana University researchers have now used this new instrument to investigate the structure of amyloid plaques (Nature Methods 10.1038/s41592-018-0053-8).
“While strictly a research tool for the foreseeable future, this technology has allowed us to see how the plaques are assembled and remodelled during the disease process,” says Gary Landreth from the Stark Neurosciences Research Institute. “It gives insight into the biological causes of the disease, so that we can see if we can stop the formation of these damaging structures in the brain.”
Brain tissue is challenging to image because it is packed with extracellular and intracellular constituents that distort and scatter light. The super-resolution nanoscope, developed by Fang Huang‘s research team at Purdue, uses adaptive optics – deformable mirrors that change shape – to compensate for aberration that occurs when light signals from single molecules travel through cells or tissue structures at different speeds.

Adaptive optics in biology
To image brain tissue, the researchers developed techniques that adjust the mirrors in response to sample depths to compensate for aberration introduced by the tissue. At the same time, they intentionally introduce extra aberration to maintain the position information carried by a single molecule.
The researchers used the nanoscope to image mice genetically engineered to develop Alzheimer’s plaques. The system reconstructs the all the tissue’s cells and cell constituents at a resolution six to 10 times higher than conventional microscopes, allowing a clear view through 30 µm thick brain sections of a mouse’s frontal cortex. The reconstructed images revealed that amyloid plaques are like hairballs, entangling surrounding tissue via small fibres that branch off waxy deposits.
“We can see now that this is where the damage to the brain occurs. The mouse gives us validation that we can apply this imaging technique to human tissue,” Landreth explains.
The collaboration is now using the nanoscope to observe amyloid plaques in human brain samples, and to study how the plaques interact with other cells and get remodelled over time.
“This development is particularly important for us as it had been quite challenging to achieve high-resolution in tissues,” says Huang. “We hope this technique will help further our understanding of other disease-related questions, such as those for Parkinson’s disease, multiple sclerosis and other neurological diseases.”
Researchers discuss how super-resolution imaging could reveal why Alzheimer’s disease starts. (Courtesy: Purdue University/Erin Easterling)