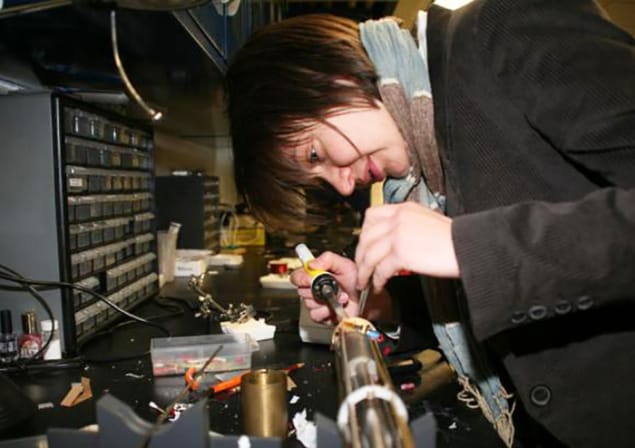
A long-sought-after phenomenon that allows superconductivity to survive even in very strong magnetic fields has been seen for the first time by an international team of physicists. The “FFLO” phase of superconductivity involves the formation of exotic quantum entities known as Andreev bound states. As well as providing further insight into superconductivity, the discovery could also further our understanding of particle physics and neutron stars, and even lead to better magnetic resonance imaging (MRI) systems.
Superconductivity and magnetism are usually sworn enemies. Superconductors will expel weak magnetic fields that would pass straight through a normal conductor, while a strong enough magnetic field will destroy superconductivity.
Conventional superconductivity occurs when vibrations in a crystal lattice allow electrons to bind together to form Cooper pairs that can flow through the lattice without resistance. The electrons in each pair have opposite values of spin angular momentum – one having spin-up while the other has spin-down. However a strong magnetic field will flip the spins of some electrons, upsetting the balance of up and down spins and so destroying the Cooper pairs and the superconductivity itself.
Mismatched electron pairs
However, in 1964 two pairs of physicists – Peter Fulde and Richard Ferrell, alongside Anatoly Larkin and Yuri Ovchinnikov – predicted that certain materials ought to superconduct, even in the presence of very strong magnetic fields. This “FFLO” state would occur as a result of mismatched electron pairs – having a finite rather than zero net angular momentum – gathering together in bands across the material, outside of which superconducting currents could still flow (see figure “Go with the FFLO: mismatched electrons”).
In the last 50 years many groups have tried to test this idea experimentally, and some have found indirect evidence for FFLO – mainly by measuring macroscopic properties of superconductors to create detailed phase diagrams of the materials. Rolf Lortz of the Hong Kong University of Science and Technology and colleagues, for example, identified a new phase between the superconducting and normal conducting phases in the organic compound κ-(BEDT-TTF)2Cu(NCS)2, which they interpreted to be FFLO and which, they found, pushed the magnetic limit for superconductivity up from 21 T to nearly 30 T.
In the latest work, Vesna Mitrović of Brown University in the US, and colleagues from Japan and the French National High Magnetic Field Laboratory (LNCMI) in Grenoble, have instead found evidence for FFLO at the microscopic scale. Their research explores the energy spectrum of a superconductor’s unpaired electrons, which have a higher energy than the paired variety. This energy gap has a single value throughout a conventional superconductor, but is predicted to vary from one region to another inside a material in the FFLO phase.
Superconducting quasiparticles
Mitrović and co-workers looked for regions within very thin sheets of κ-(BEDT-TTF)2Cu(NCS)2 where the energy gap goes to zero. These are regions where paired and unpaired electrons have the same energy, and where it is therefore energetically possible for unpaired electrons to exist. These unpaired electrons are best thought of as “quasiparticles”, which exist in complicated quantum superpositions with everything around them, and, unlike normal electrons, can superconduct. Specifically, the researchers looked for quasiparticles known as Andreev bound states, which resemble normal electrons whose spins point in the direction of an applied magnetic field.
The experiment was carried out at the LNCMI, where nuclear magnetic resonance (NMR) was used to confirm two expected properties of Andreev bound states – and therefore the presence of the FFLO phase. The first, and most important, involved measuring the time that it took for electrons to flip their spin when exposed to powerful magnetic fields, a characteristic that reflects the energy spectrum of electrons across the sample. The second property required measuring the distribution of spins within the material.
“Other groups have carried out impressive and important work, showing that in a high magnetic field you go into a new state,” says Mitrović. “But they could not tell what this state looked like. The purpose of our experiment was to look, and what we see is actually quite striking.” She adds that the work might prove to be important outside of condensed-matter physics, because it could help particle physicists to identify a form of superconductivity that involves quarks with unbalanced flavour, and in astrophysics might explain how neutron stars can exhibit superconductivity while at the same time generating enormous magnetic fields.
Better MRI systems
Lortz says that the research provides “important information of a different kind” to that obtained by his group. He adds that, in principle, it could lead to the creation of more powerful superconducting magnets for MRI systems because the superconducting state persists to higher fields. While κ-(BEDT-TTF)2Cu(NCS)2 is not suitable for making magnets, Lortz adds that the FFLO phase might be observed in more appropriate materials in the future.
Ted Forgan of the University of Birmingham, who has looked for FFLO in the superconductor CeCoIn5, says that the results look “pretty convincing”. But he points out that NMR, while providing microscopic data, does not show spatial variation directly. “Maybe high-field scanning tunnelling microscopy or spectroscopy could show a spatially modulated state,” he says.
The research is described in Nature Physics.