
Tensile cracks in brittle elastic materials can spread faster than the speed of sound – and faster than the laws of classical fracture mechanics say is possible. The new fracture mode was discovered by a team at the Racah Institute of Physics at the Hebrew University of Jerusalem, Israel, and could overturn traditional pictures of what happens when things break.
Materials fail when cracks form and propagate within them. Classical fracture mechanics says that these tensile cracks should move in a way that dissipates the elastic energy that builds up within a point-like zone at their tips. One consequence of this is that a classical tensile crack cannot travel faster than the Rayleigh wave speed, cR, which is related to the material’s shear-wave velocity and how much it deforms under a load (its Poisson ratio). However, Meng Wang, Songlin Shi and Jay Fineberg have now found that some cracks do not obey this rule. Instead, they smoothly accelerate to near-supersonic speeds.
“We are rather excited by this discovery,” Fineberg tells Physics World. “The very existence of these ‘supersonic failure modes’ calls into question the fundamental physical assumptions that underpin our current understanding of the fracture process. It is not that the established framework is wrong, but it implies that there is not a unique ‘set of rules’ that guide fracture.”
Cracks move unexpectedly quickly
In the new work, which is detailed in Science, Fineberg and his colleagues studied brittle gels that are “neo-Hookean”, meaning that they have a nonlinear relationship between applied stress and strain. Using soft materials like these slows the speed of crack propagation by about three orders of magnitude, allowing the team to observe crack dynamics with fast, high-resolution cameras while making precise, real-time measurements on the strain fields surrounding the crack tips. Such measurements would have been impossible in a material such as glass, Fineberg stresses.
The team’s previous work had indicated that cracks in these brittle gels do not behave any differently from cracks in standard brittle materials. This time, however, when they uniformly stretched sheets of the material and introduced a small cut to create an initial crack, the velocities of the cracks hit speeds never previously documented, with the fastest moving about 30% faster than the speed of sound.
These observations contradict earlier studies, both theoretical and experimental, showing that cracks cannot propagate faster than sound. This is because sound speed reflects how quickly mechanical energy can move from one part of a material to another – something that must occur for it to crack.
The researchers say their observations must therefore indicate the presence of “supershear” dynamics that are governed by different principles than those guiding classical cracks. Notably, the new mode of tensile fracture does not occur randomly. Instead, it is triggered at critical strain levels that depend on the material. Such effects had been suggested theoretically nearly two decades ago by Michael Marder at the University of Texas at Austin in the US, but “since they were so different to the accepted description of fracture, they were not taken too seriously by many in the field,” Fineberg explains. “The new experiments irrefutably demonstrate that such modes of fracture both can and do exist – and under what conditions.”
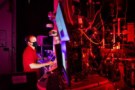
Fatigue-generated cracks fuse back together in metals
A new mode of fracture
Fineberg adds that he and his colleagues came upon their results by accident while trying to study an entirely different phenomenon. “The challenge was, after we had convinced ourselves that these initially unexpected effects were real, to try and obtain a physical picture of what determines the transition to this new type of fracture,” he says. “This involves relating what happens in the singular region near the crack tip to its macroscopic (large-scale) behaviour.”
The team is now working to characterize the new mode of facture it unearthed. “In parallel we will compare our observations to both Michael Marder’s original theoretical description and to new theory and calculations,” Fineberg says. “We are only just at the beginning of understanding the effects we have observed.”