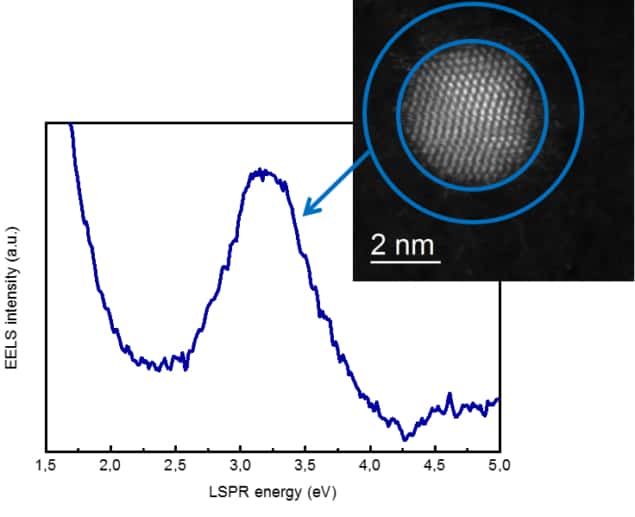
The physical properties of metal particles change the smaller they get and new phenomena such as localized surface-plasmon resonance, or LSPR, appear. The LSPR (which is responsible for the colour in nanocolloidal solutions or in some stained-glass windows) is a collective excitation of electrons and it can be tuned over a large spectral range depending on the material, its size and shape. Experiments to study this effect have so far produced conflicting results, however, but researchers in France say they may now be able to explain these earlier contradictions. Their work is not only important for our fundamental understanding of how quantum mechanics affects classical effects like LSPR, but it might also help advance applications such as photocatalysis, biomedical imaging or sensing, all of which exploit LSPR.
Surface plasmons are collective excitations of electrons at the surface of a metal that interact very strongly with light. They are thus interesting for technological applications as an interface between photonics and electronics. The light-matter interaction is strongest at the plasmon-resonance frequency, which is defined by the size and shape of an object and its charge density, and surface plasmons can generate strong electric fields at this frequency.
Inconsistent results so far
Research on LSPR so far has been beset with inconsistencies, however. “Some previous experiments to study LSPR reported on a particle-size-dependent plasmon shift, for example, but others did not,” explains Matthias Hillenkamp of the University of Lyon, who led this research effort. “We wanted to find out whether, and how, this shift is determined by the local environment of the nanoparticles.”
And this is exactly what the researchers did – by showing that in very small nanoparticles (<10 nm in diameter), the surface plasmon resonance is indeed influenced by quantum effects, but that these are sometimes quenched depending on the environment.
Electron spill-out and d-orbital electron screening
“The most important of these effects are electron spill-out (in which the electronic wavefunction extends beyond the radius of a particle) and reduced d-orbital electron screening at the particle surface,” says Hillenkamp. “These two effects are the opposite of each other: the first shifts the plasmon resonance towards the red part of the electromagnetic spectrum while the second shifts it to the blue. The actual spectral position of the resonance is a balance between the two, and it is affected by the environment.”
The researchers, reporting their work in Nature Physics 10.1038/s41567-018-0345-z, obtained their results by studying ensembles of silica-embedded silver nanoparticles using optical absorption spectroscopy, which is the most widely employed technique to investigate such particles in this context. They looked at particles ranging in size down to less than 1 nm diameter, which is where quantum size effects are expected, and found that all but the very smallest particles strongly absorb light at a bandwidth of 2.95 eV, irrespective of their size.
For nanoparticles in the same size range, they also performed single particle electronic spectroscopy in an electron microscope. This technique allows to correlate particle size and shape with the plasmon response of one single particle, down to sizes below 2 nm in diameter (which is just a little more than 100 atoms).
Semi-quantitative model
“At first, our results again seemed contradictory, but only after identifying that the electron beam alters the local environment, were we able to explain our findings with the help of a semi-quantitative model that takes into account the electron spill-out and reduced d-orbital electron screening effects mentioned earlier,” Hillenkamp tells Physics World.
The team, which includes researchers from the CNRS, Paris-Sud University and Aix-Marseille University, says that is now extending its investigations to more complex systems such as nanoalloys and to different physical effects like the volume plasmon. “This has been known for a long time but it is difficult to study since it is impossible to excite with light,” says Hillenkamp.