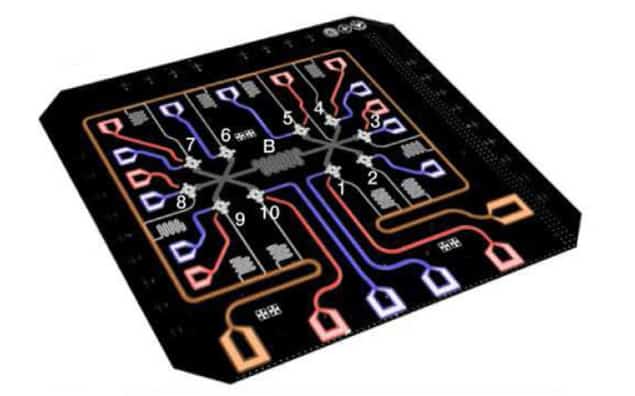
A group of physicists in China has taken the lead in the race to couple together increasing numbers of superconducting qubits. The researchers have shown that they can entangle 10 qubits connected to one another via a central resonator – so beating the previous record by one qubit – and say that their result paves the way to quantum simulators that can calculate the behaviour of small molecules and other quantum-mechanical systems much more efficiently than even the most powerful conventional computers.
Superconducting circuits create qubits by superimposing two electrical currents, and hold the promise of being able to fabricate many qubits on a single chip through the exploitation of silicon-based manufacturing technology. In the latest work, a multi-institutional group led by Jian-Wei Pan of the University of Science and Technology of China in Hefei, built a circuit consisting of 10 qubits, each half a millimetre across and made from slivers of aluminium laid on to a sapphire substrate. The qubits, which act as non-linear LC oscillators, are arranged in a circle around a component known as a bus resonator.
Initially, the qubits are put into a superposition state of two oscillating currents with different amplitudes by supplying each of them with a very low-energy microwave pulse. To avoid interference at this stage, each qubit is set to a different oscillation frequency. However, for the qubits to interact with one another, they need to have the same frequency. This is where the bus comes in. It allows qubits to transfer energy from one another, but does not absorb any of that energy itself.
“Magical interaction”
The end result of this process, says team member Haohua Wang of Zhejiang University, is entanglement, or, as he puts it, “some kind of magical interaction”. To establish just how entangled their qubits were, the researchers used what is known as quantum tomography to find out the probability of detecting each of the thousands of possible states that this entanglement could generate. The outcome: their measured probability distribution yielded the correct state on average about two thirds of the time. The fact that this “fidelity” was above 50%, says Wang, meant that their qubits were “entangled for sure”.
According to Shibiao Zheng of Fuzhou University, who designed the entangling protocol, the key ingredient in this set-up is the bus. This, he says, allows them to generate entanglement “very quickly”.
The previous record of nine for the number of entangled qubits in a superconducting circuit was held by John Martinis and colleagues at the University of California, Santa Barbara and Google. That group uses a different architecture for their system; rather than linking qubits via a central hub they instead lay them out in a row and connect each to its nearest neighbour. Doing so allows them to use an error-correction scheme that they developed known as surface code.
High fidelity
Error correction will be vital for the functioning of any large-scale quantum computer in order to overcome decoherence – the destruction of delicate quantum states by outside interference. Involving the addition of qubits to provide cross-checking, error correction relies on each gate operation introducing very little error. Otherwise, errors would simply spiral out of control. In 2015, Martinis and co-workers showed that superconducting quantum computers could in principle be scaled up, when they built two-qubit gates with a fidelity above that required by surface code – introducing errors less than 1% of the time.
Martinis praises Pan and colleagues for their “nicely done experiment”, in particular for their speedy entangling and “good single-qubit operation”. But it is hard to know how much of an advance they have really made, he argues, until they fully measure the fidelity of their single-qubit gates or their entangling gate. “The hard thing is to scale up with good gate fidelity,” he says.
Wang says that the Chinese collaboration is working on an error-correction scheme for their bus-centred architecture. But he argues that in addition to exceeding the error thresholds for individual gates, it is also important to demonstrate the precise operation of many highly entangled qubits. “We have a global coupling between qubits,” he says. “And that turns out to be very useful.”
Quantum simulator
Wang acknowledges that construction of a universal quantum computer – one that would perform any quantum algorithm far quicker than conventional computers could – is not realistic for the foreseeable future given the many millions of qubits such a device is likely to need. For the moment, Wang and his colleagues have a more modest aim in mind: the development of a “quantum simulator” consisting of perhaps 50 qubits, which could outperform classical computers when it comes to simulating the behaviour of small molecules and other quantum systems.
Xiaobo Zhu of the University of Science and Technology of China, who was in charge of fabricating the 10-qubit device, says that the collaboration aims to build the simulator within the next “5–10 years”, noting that this is similar to the timescale quoted by other groups including the one of Martinis. “We are trying to catch up with the best groups in the world,” he says.
The research is reported on the arXiv server.