A decade after the creation of the first Bose-Einstein condensate, research into the properties of ultracold atoms is about to enter a new era. Peter Rodgers reports
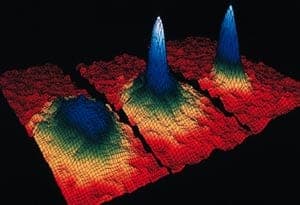
At 10.54 a.m. on Monday 5 June 1995 a group of physicists at the JILA laboratory in Boulder, Colorado, created something that had never been seen before – a Bose-Einstein condensate made of atoms. Eric Cornell, Carl Wieman and colleagues had made a new state of matter by cooling a gas of rubidium-87 atoms to a temperature of just 170 nK. This was so cold that the de Broglie wavelength of the atoms was comparable with the distance between them, causing the atoms to condense into the same quantum ground state. The breakthrough in Boulder kick-started an intense period of research into the properties of ultracold atoms that continues to this day, and has implications for topics as diverse as superfluidity and the early universe.
Within months of the first observation, Bose-Einstein condensation had also been seen in lithium-7 by Randy Hulet’s group at Rice University in Texas and in sodium-23 by Wolfgang Ketterle and co-workers at the Massachusetts Institute of Technology (MIT). And by the time Cornell, Ketterle and Wieman shared the 2001 Nobel Prize for Physics, condensates had also been seen in hydrogen, helium and potassium-41. Moreover, a whole new frontier of research was opening up – the study of ultracold Fermi gases.
“What has happened in the past year with Fermi gases has only been topped by the discovery of the first condensates in 1995,” says Ketterle. “But this is just the beginning – research into Fermi gases is now connecting to a long list of intellectual challenges in condensed-matter physics, such as superfluidity, superconductivity, magnetism and so on.”
Fermions and bosons
All atoms, indeed all particles, fall into one of two fundamental classes depending on the value of their intrinsic angular momentum or “spin” in quantum units – atoms with spins of 1/2, 3/2 and so on are fermions, whereas those with integer spins are bosons. The difference becomes clear at very low temperatures: large numbers of bosons can collapse into the same quantum state to form a condensate, whereas the Pauli exclusion principle prevents fermions from doing the same. Rather, as a Fermi gas is cooled, more and more of the lowest energy levels fill up until all the levels below the Fermi energy are occupied. The first such “degenerate” Fermi gas was produced in potassium-40 by Deborah Jin and Brian DeMarco in Boulder in 1999.
Last year Jin and colleagues went a step further by producing the first Fermi condensate, again in potassium-40 (see “Fermionic first for condensates”). A Fermi condensate might seem like a contradiction in terms but it is not: two fermionic atoms can join forces to form a molecule or the atomic equivalent of a Cooper pair, just like electrons form pairs in the Bardeen-Cooper-Schrieffer (BCS) theory of superconductivity. The molecules and the pairs both behave as bosons because they both have integer spin.
Most of these experiments rely on a phenomenon known as a Feshbach resonance to control the interactions between the atoms. First demonstrated in condensates by Ketterle and co-workers in 1998, the Feshbach resonance has become an essential technique in many experiments on ultracold atomic gases, including the condensation of molecules. “In the early days of BEC the strength of the interactions between the atoms was fixed,” says Rudolf Grimm of the University of Innsbruck in Austria. “The discovery of Feshbach resonances changed this completely and led to a new world of experiments.”
Fermi gases have proved to be a very rich source of new results. Indeed, since quarks, electrons and all the fundamental matter particles in nature are fermions, degenerate Fermi gases have the potential to be even more interesting than their bosonic counterparts. However, this comes at a cost. “In general, fermions are more challenging to work with than bosons,” says Randy Hulet at Rice, “due to the difficulty in cooling them and measuring their temperature. These challenges make any experiment more difficult.”
One of the problems is that collisions between atoms play a central role in the cooling of atoms, but the exclusion principle prevents identical fermions getting too close to one another. One way to overcome this problem is to mix bosons and fermions in the same trap and rely on collisions between the two species – which are not forbidden by the exclusion principle – to do the cooling. This approach is known as sympathetic cooling.
However, the absence of collisions can be an advantage in some experiments, according to Massimo Inguscio of the University of Florence in Italy. When using beams of ultracold atoms to measure small forces in interferometer experiments, for instance, fermionic atoms give better results because collisions between bosons reduce the precision of the measurements.
Building bridges
Most researchers working on ultracold atoms believe that the next big goals in the field are to make connections with other areas of physics. The observation of superfluidity in a Fermi gas is widely seen as the next milestone, and it is common knowledge in the community that Ketterle and co-workers have just seen conclusive evidence for this in the form of quantized vortices in a rotating gas of lithium-6 atoms. This should confirm less direct evidence for superfluidity seen at a number of other labs.
Another challenge is to explore the cross-over between the BEC region in which molecules are condensed and the BCS regime in which pairs of atoms are condensed (see “Superfluidity in Fermi gases”). “A number of experiments are under way but we need some better theory of the phenomena to guide them,” says Keith Burnett, a theorist at Oxford University in the UK.
The race is also on to explore the properties of a Fermi gas trapped in an optical lattice – a 3D landscape in which the energy varies to form a perfect lattice of peaks and troughs. If the lasers producing the lattice are intense enough, atoms can be trapped at the peaks (or troughs). Such an experiment would basically reproduce what happens inside a crystal, with the atoms playing the role of the electrons and the lattice representing the ions. The advantage of the ultracold approach, however, is that it is possible to control the interactions between the atoms, which is impossible with the electrons in an ordinary solid (see “Quantum gases in optical lattices”).
In late 2001 Immanuel Bloch, then based in Munich, and co-workers managed to trap ultracold rubidium atoms in an optical lattice and observe phase transitions between the normal superfluid state of a Bose condensate and a state known as a Mott insulator. However, to date only two groups – Inguscio and co-workers in Florence and Tilman Esslinger’s group at ETH Zurich – have managed to repeat this feat with a Fermi gas.
“The next steps will be to understand the influence of atom-atom interactions in the lattice,” says Esslinger. “Various phases that have been predicted by theorists may then become available in the experiment. From the experimental point of view, the challenge is to reach low enough temperatures and to extract the information from the experimental observables.”
Thinking outside the box
While most researchers are looking to the parallels with condensed-matter systems, a small number are moving in a completely different direction. For instance, some groups are exploring the use of condensates to make quantum computers that can perform certain tasks much faster than is possible on a classical computer. However, there is a lot of competition from other approaches. “Condensates have lots of potential quantum bits,” says Chris Monroe of the University of Michigan, “but it remains very difficult to address and control individual neutral atoms at the same level as ions.”
Other groups are hoping to show that the electron has a small electric dipole moment, as predicted by certain extensions of the Standard Model of particle physics. These experiments currently involve making extremely precise measurements on atoms such as caesium and thallium, or on dipolar molecules such as ytterbium fluoride.
Another line of research involves experiments in which the Feshbach resonance is used to make the scattering length – which describes the interactions between the atoms – much longer than the average distance between them. Such gases are said to be strongly interacting. In 2002 John Thomas and co-workers at Duke University in North Carolina produced the first Fermi gas that was both strongly interacting and degenerate. However, when they released the gas from the trap – which was shaped like a cigar – they noticed something unusual: it expanded more rapidly in the narrow direction than in the long direction.
It then emerged that similar behaviour had been seen in experiments built to produce a quark-gluon plasma – a state containing free quarks and gluons that last existed just a fraction of a second after the Big Bang (see “Liquid universe hints at strings”).
“The basic connection between a quark-gluon plasma and our strongly interacting Fermi gas is that they both obey nearly perfect hydrodynamics, where perfect means zero damping and zero viscosity,” says Thomas, who has recently started working with Ed Shuryak, a nuclear theorist at Stony Brook University, on these topics. More generally, Thomas sees strongly interacting Fermi gases as a way of testing the theoretical methods that are used in other areas of physics where strong interactions dominate. Indeed, a recent paper on string theory referenced the Duke group’s 2002 experiment.
And this is not the only connection between ultracold gases and strings. Only last month a group of theorists at Utrecht University in the Netherlands proposed that superstrings could be made in the laboratory by trapping an ultracold cloud of fermionic atoms inside a vortex in a Bose condensate (see “A recipe for making strings in the lab”). Given the progress that has been made over the past 10 years, it should surprise nobody if someone actually manages to do the experiment.
Where are they now?
When Eric Cornell, Wolfgang Ketterle and Carl Wieman shared the Nobel prize in 2001, they became three of the best-known physicists in the world. Cornell and Ketterle remain very active in condensate research, although Cornell was diagnosed with necrotizing fasciitis last October and subsequently had to have his left arm and shoulder amputated. He returned to his lab part-time in April, with plans to search for the dipole moment of the electron. “The doctors tell me I am lucky to be alive,” he said, “and I am much inclined to agree with them.”
Wieman, meanwhile, claims to have retired from thinking about big goals in physics. “Nowadays I am satisfied to work on physics problems that seem interesting to me but are not aimed at big goals,” he says. “However, I am thinking about big goals in science education and how to reach them. My main conclusion is that current science education is failing badly at achieving the needs of the 21st century – it needs to be effective and relevant to a large fraction of the population, and not just the small fraction going into science.”
Wieman, who was named US professor of the year last year for “his unwavering dedication to undergraduate teaching”, is convinced that science education requires a scientific approach. “When practices based on good data, disseminating and duplicating what works and so on are combined with effective uses of technology, it is possible to vastly improve science education without it requiring a great deal of additional cost or faculty time,” he says.
And what happened to the graduate students and postdocs who worked on the original BEC experiments at Boulder and MIT? Michael Anderson, the first author on the Boulder paper, joined a company called Meadowlark Optics, where he worked his way up to be vice-president in charge of R&D and manufacturing.
“I worked there until the autumn of 2001, when the bottom fell out of the telecoms market,” he recalls. “Developing reliable manufacturing processes and product improvements was quite challenging technically. However, I found learning about business and the ‘how to win friends and influence people’ side of the job especially challenging – business skills are something that a physics education does not prepare you for!” After considering a career as a cabinet maker, Anderson recently set up his own company, Vescent Photonics, to make sensors based on a new type of diode laser.
Anderson’s co-workers also followed careers in industry. Jason Ensher is a systems engineer for Ball Aerospace & Technologies, while Mike Matthews currently works for 3M in Texas. “It was a great experience to attend the Nobel-prize ceremony in 2001,” says Matthews, “but I don’t regret leaving the field of BEC specifically. However, I do miss the almost boundless curiosity and the energy that you find at a place like JILA.”
Two of the co-authors on the MIT paper – Ken Davies and Marc-Oliver Mewes – are management consultants, while a third, Michael Andrews, has worked for Lucent and start-ups. The other three have remained in academic research: Dallin Durfee and Dan Stamper-Kurn have faculty positions at Brigham Young University and the University of California at Berkeley, respectively; while Klaasjan van Druten is project leader at the University of Amsterdam.
Further reading
Physics World and PhysicsWeb have published many articles on Bose-Einstein condensates and degenerate Fermi gases. See:
Chromium condensate makes its mark PhysicsWeb March 2005
Fermi gas goes superfluid PhysicsWeb July 2004
Fermi gases approach superfluid regime PhysicsWeb April 2004
Quantum gases in optical lattices Physics World April 2004
Fermionic first for condensates Physics World March 2004
Fermionic condensate makes its debut PhysicsWeb January 2004
Condensates enter new era PhysicsWeb November 2003
Bose-Einstein condensates break temperature record PhysicsWeb September 2003
Quantum gases come of age Physics World September 2003
Ytterbium joins the condensates PhysicsWeb July 2003
Ultra-cold Fermi gases enter new regime PhysicsWeb December 2002
Caesium condensate makes its debut PhysicsWeb December 2002
Caesium joins the condensates PhysicsWeb October 2002
Condensate cracks Fermi gas PhysicsWeb September 2002
Condensates on crest of a wave Physics World July 2002
Tantalizing evidence for molecular condensation PhysicsWeb May 2002
Condensates get longer lives PhysicsWeb May 2002
A Fermi gas of atoms Physics World April 2002
New look for Bose condensates PhysicsWeb January 2002
Condensates reap Nobel reward Physics World November 2001
Condensate control could lead to ‘atom circuits’ PhysicsWeb October 2001
Taming light with cold atoms Physics World September 2001
Condensates create Josephson arrays PhysicsWeb August 2001
Bose-Einstein condensation bursts out PhysicsWeb July 2001
Triple first for Bose condensates Physics World May 2001
Quantum entanglement spreads to Bose condensates PhysicsWeb June 2000
Molecules made in a Bose-Einstein condensate PhysicsWeb February 2000
Condensates in a whirl PhysicsWeb October 1999
Fermions go degenerate PhysicsWeb September 1999
Atom lasers Physics World August 1999
Matter makes waves PhysicsWeb March 1999
Hydrogen: a quantum gas at last Physics World February 1999
Putting the quantum brakes on light PhysicsWeb February 1999
Amplification of matter waves PhysicsWeb February 1998
Bose-Einstein condensation Physics World March 1997
Summaries of the following Physics World articles appear on PhysicsWeb
Ultracold fermion race is on Physics World August 1999 p19
Bose condensates make quantum leaps and bounds Physics World August 1999 pp37-42
Quantum fluids create a stir in the lab Physics World January 2000 pp20-21
Amplifier means gains for atom optics Physics World March 2000 p24
Chaos gives quantum tunnelling a hand Physics World September 2001 pp24-25
Bosons help to beat the Fermi pressure Physics World November 2002
Superfluidity in Fermi gases Physics World March 2005
The following articles only appear in the print version of Physics World
Magnetic traps hit a new low Physics World July 1995 p21
Atoms lose their identity Physics World August 1995 p21
Bose breakthrough Physics World December 1995 p8
Flipping the switch on an atom laser? Physics World October 1996 p18
Hydrogen condensate is go Physics World August 1998 p5
Condensation in a spin Physics World March 1999 p5
A double first close to absolute zero Physics World April 2001 p3
Condensates made easy Physics World August 2001 p3