Philip Ball reviews Einstein’s Fridge: the Science of Fire, Ice and the Universe by Paul Sen
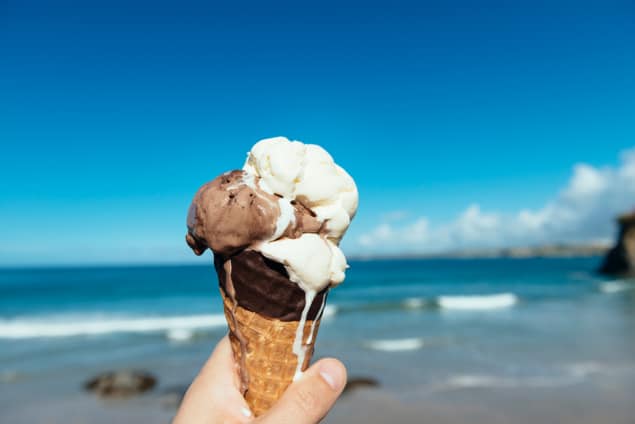
Like many science writers, I have often adopted the conceit that quantum mechanics and general relativity are our two principal (if incompatible) theories of the physical world. With his superb new book Einstein’s Fridge: the Science of Fire, Ice and the Universe, documentary filmmaker Paul Sen has made me doubt that this is the right way to express it. Those two theories might be better positioned as the terms of engagement with the universe: relativity describing the backdrop of space–time, and quantum theory giving a fine-grained account of the stuff within it. But to explain what we actually see happening amid all the teeming particles, we are better served by the subject of Sen’s book: thermodynamics, in all its guises.
Einstein’s Fridge offers an accessible and crystal-clear portrait of this discipline’s breadth, largely told through its history. Beginning as an attempt to understand and improve the machinery of the Industrial Revolution, thermodynamics soon provided the most profound of what physicists today call no-go theorems – statements about what is physically impossible – in the form of the first two laws of thermodynamics. First, energy is conserved in the universe; second, in all that comes to pass, some energy is unavoidably dissipated into a form that cannot be used to do work. From those ingredients, 19th-century scientists realized that we can make deductions about the beginning of the universe (it must have had an unlikely, low-entropy configuration) and its end (in a “heat death” of useless energetic uniformity).
Thermodynamics tells us everything from the prosaic (why ice cream melts), to the global (what powers the engine of the world’s climate system) and even the cosmic (why the Big Bang has left a pervasive microwave background hum). It holds the key to life’s mysteries – how living organisms seem to (but in fact do not) evade the second law, which demands increasing entropy. It undergirds the theories of information and computation, for example via mathematician Claude Shannon’s definition of information entropy. It makes startling predictions about black holes, such as Stephen Hawking’s proposition that they slowly evaporate, which general relativity alone could not reveal. Perhaps most profoundly, the first law (conservation of energy) connects to the deep symmetry properties of physical theory, as German mathematician Emmy Noether proved: energy conservation follows from the time-symmetric behaviour of fundamental physical laws, just as momentum conservation is related to their translational invariance in space.
We too easily forget how central thermodynamics was to the evolution of quantum theory in the first place. It was only by assuming a convenient heuristic about microscopic vibrations that Max Planck was able, in 1900, to develop a theory for how hot bodies emit radiation – specifically, he assumed the vibrations are quantized. It was Einstein, of course, who was bold enough to take that idea literally. Sen reminds us how much of Einstein’s early work was rooted in the microscopic picture of thermodynamics developed by James Clerk Maxwell, Ludwig Boltzmann and others. Einstein used the bulk, aggregate properties of matter to estimate molecular dimensions. Most famously, he provided the theory of Brownian motion that underpins diffusion and enabled the French physicist Jean Perrin to adduce compelling evidence for the reality of atoms in 1908.
Einstein’s fridge itself, which was a rare diversion he took into applied physics, developed in conjunction with fellow physicist Leo Szilard, was therefore of a part with his enthusiasms. Motivated by accounts of deaths from leaky, toxic refrigerants, the duo designed a fridge that had no moving parts and so would be less liable to malfunction. Their idea was developed into a prototype by the German company AEG but fell by the wayside when a non-toxic coolant called Freon was developed by General Motors in 1930. (There has recently been renewed interest in the Einstein–Szilard design.)
Szilard, meanwhile, helped to unravel the conundrum posed by Maxwell in his effort to “pick a hole” in the second law by invoking a tiny being (dubbed a demon by Lord Kelvin) that could selectively separate hot gas molecules from cold. Szilard showed how this scenario could be simplified and automated to eliminate the mysteries of the demon’s cogitation. But it was not until the 1960s that the problem was solved, in a manner that connected thermodynamics to computation. It turns out that the second law is protected by the demands placed on a memory for recording molecular motions: there is an unavoidable entropic cost of erasing information, which sets a fundamental limit on the energy efficiency of computation.
Einstein’s Fridge wanders widely while never losing its connection to the central theme
Sen explains all this with admirable clarity – I particularly welcomed his account of how Kelvin’s temperature scale works, and why it imposes a lower limit on how cold things can get. Einstein’s Fridge wanders widely while never losing its connection to the central theme, and shows how the science that was begun by the 18th- and 19th-century scientists Sadi Carnot, Count Rumford and Rudolf Clausius really does shore up most of modern physics.

‘Quintuple point’ material defies 150-year-old thermodynamics rule
In this regard, Sen could have ranged even further afield. Thermodynamics, and its modern incarnation of statistical physics, is really the science of emergent behaviour in interacting many-body systems. This is why its core concepts – such as phase transitions, critical points, renormalization and stochasticity – recur throughout many branches of physics. They appear in the quantum-chromodynamic descriptions of hadrons; the theories of superconductivity and strongly correlated electrons; the mechanisms of living cells (where for example phase separation has recently been recognized as literally vital); and even the behaviour of crowds, traffic and animal swarms.
This makes it the most fundamental description we have of what transpires in the world at all scales, both in nature and in our artificial and technological systems. Sen is right to say that it is “arguably the most useful and universal scientific theory ever conceived”. All the stranger, then, that it has received so little attention in the popular sphere, that it is given such piecemeal treatment in education, and that it has such a dull reputation. (Like Sen, I found it rather tedious and narrow as a callow undergraduate.) All the better, then, that there is now a splendid antidote to that neglect.
- 2021 William Collins £20hb 320pp