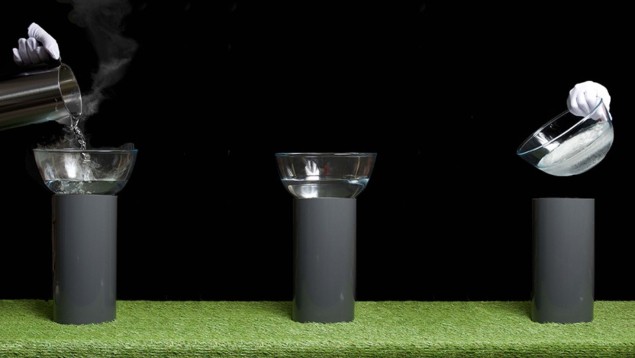
A consequence of the second law of thermodynamics is that heat spontaneously flows from hot to cold, not the other way around. But now researchers in Switzerland have now shown that, if two reservoirs at different temperatures are connected using a passive thermoelectric element, “thermal inertia” can allow the hot reservoir to cool down to below the temperature of the cold reservoir. While this does not violate the second law, thermoelectic materials available today are not good enough for the effect to be exploited in practical devices. However, the researchers believe it could one day be used in refrigeration.
The thermoelectric effect is a well-known phenomenon whereby some materials convert a temperature difference into a potential difference. Heating one end of a metal, for example, causes the excited electrons at the hot end to diffuse towards the cold end. The effect also works in reverse: applying an electric current to a thermoelectric material leads creates a temperature gradient. This is the basis of thermoelectric coolers, which are widely used in computers, hotel minibars and other situations where the compressor required by a standard refrigerator would be unfeasible.
In thermoelectric coolers, heat flow from cold to hot is driven by external energy input. In this latest research, Andreas Schilling and colleagues at the University of Zurich dispensed with an external power supply. They started from two reservoirs at different temperatures. If these were placed in thermal contact, heat would simply flow from the hot reservoir to the cold until the two reached thermal equilibrium. Instead, however, they connected them electrically through a thermoelectric element, allowing the heat flow to drive an electric current. Crucially, they also added an electrical inductor.
Going with the flow
Inductors are among the most basic electrical components, usually comprising just a coil of wire. As current flows through the inductor, a magnetic field is induced in the coil. If the current is increased, the inductor draws energy from the current into the magnetic field. If the current is reduced, the inductor returns this energy: “With an inductor there is a kind of electrical inertia,” explains Schilling, “Once the current is flowing, it wants to stay flowing.”
When the two reservoirs are initially connected, therefore, the inductor resists the increase in electric current induced by the thermoelectric effect. As the temperature difference drops, however, the induced current also drops, and the inductor resists this drop. When the temperature difference reaches zero, the electrical inertia allows current to keep flowing. The circuit now behaves as a thermoelectric cooler, continuing to push heat from the hot reservoir to the cold reservoir even though the “hot” reservoir is now colder. The electrical inertia therefore causes a “thermal inertia” – a heat current that requires energy to stop. Eventually, the temperature difference becomes sufficiently large to induce a current back the other way, and the process is repeated. This leads to temperature oscillations between the two reservoirs, which are eventually damped by losses in the circuit.
Despite the repeated heat flow from cold to hot in both directions, the researchers calculated the overall entropy of their system always increases, as required by the second law. The key is that, when the two reservoirs are the same temperature, this temperature is slightly lower than the mean temperature of the two heat baths at different temperatures. “When the average temperature goes down by maybe 1%, this seemingly lost energy is stored temporarily in the inductor,” explains Schilling. The return of this seemingly negligible energy allows the total entropy of the two heat baths to increase, even as heat is driven from cold to hot.
Perfect thermoelectric needed
The researchers heated the hot reservoir to 104 °C, before connecting it to a thermoelectric material at 22 °C. In principle, the laws of thermodynamics allow the hot reservoir to be cooled to -47 °C – allowing boiling water to be cooled to ice simply by connecting it to a room-temperature thermoelectric. This would require a perfect thermoelectric material, however, and current thermoelectrics are nowhere near this. In practice, the largest cooling the researchers achieved was less than 3 °C below 22 °C. Nevertheless, says Schilling, thermoelectric development is one of the most active areas in materials science because of their potential to produce compressor-free commercial refrigerators.
“Fundamentally, I think it’s very interesting,” says Greg Walker of Vanderbilt University in Nashville, Tennessee. He is more sceptical about applications, however: “I’m not sure how you would build a company around it and not really sure what you would do with it.”
The research is described in Science Advances.