Physicists in the US have taken a significant step towards nanometre-scale electronics by creating the first transistor made from a single atom. A similar device based on a single divanadium molecule – which consists of two vanadium atoms – has also been built by a second US team. The researchers are optimistic that the transistors will prove to be rich sources of exotic electronic effects, and that their achievements will stimulate research that could lead to the development of practical nanoelectronics systems.
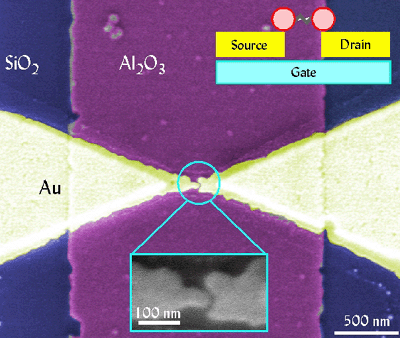
Transistors are ubiquitous in today’s silicon-based microelectronics industry. The three-terminal devices act as switches because the current flowing from the source to the drain electrodes can be turned on and off by a voltage applied to the gate electrode. Transistors are usually made from layers of different semiconductors, and the current that flows through them consists of around a billion electrons. But in the new devices, electrons can hop one at a time from the source to the drain electrodes across a ‘bridge’ made from the single atom or molecule.
Paul McEuen and co-workers at Cornell University and the University of California at Berkeley based their transistor on a single cobalt atom suspended in an organic compound (J Park et al 2002 Nature 417 722). They deposited a gold wire about 10 nm thick onto a silicon substrate and then coated it with the complex. The team then made a gap in the wire about 1 nm wide, so that the exposed tips formed source and drain electrodes. Molecules of the organic complex then slipped into this gap, taking a cobalt atom with them. A layer of silicon dioxide insulated the gap region from the silicon substrate, which acted as the gate electrode.
In a similar way, Hongkun Park and colleagues at Harvard University and the University of California at Berkeley trapped divanadium molecules between gold electrodes – also 1 nm apart – and used aluminium oxide to isolate the gap region from their silicon gate electrode (W Liang et al 2002 Nature 417 725).
Both teams then measured the current flowing from the source to the drain as they varied the voltage between these electrodes for a range of gate voltages. For each device, the researchers found that the current flowed only at certain gate voltages, which were related to the energy that an electron needs to hop on – or off – the bridging atom or molecule.
This behaviour is the hallmark of a single-electron transistor, but both groups also found that more electrons took part in the current flow when they placed their devices in a magnetic field. The researchers believe that this phenomenon arises from the ‘Kondo effect’, in which a single magnetic entity – such as a cobalt atom or a divanadium molecule – interacts with the electrons in the non-magnetic material around it.
McEuen’s and Park’s teams also found that the exact electronic properties of their transistors could be tuned by changing the chemical make-up of the organic compounds. This determines the quality of the electrical connection between the atom or molecule and the electrodes.
Both groups are now investigating these effects in transistors based on other atoms and molecules. “We are already working on half a dozen different single-molecule transistors that incorporate different molecules, including single-molecule magnets,” Park told PhysicsWeb.