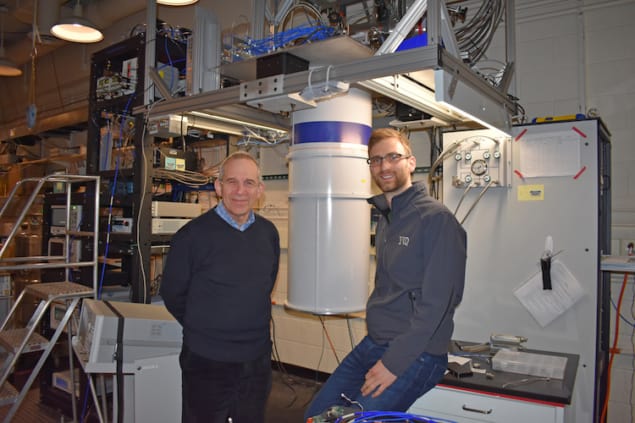
Is it possible to know if a quantum jump is about to occur? Researchers have been asking themselves this question for a long time, and the answer is a resounding “yes”, according to a new study by a team at Yale University in the US. As well as being of great importance for fundamental physics studies, the result, obtained by measuring the flight of a superconducting artificial three-level atom when it is excited by a beam of microwaves, might even be useful for correcting errors in quantum computing in the future.
It was Niels Bohr in 1913 who first put forward the concept of quantum jumps, which are the sudden transitions of a tiny object (such as an electron, molecule, or atom) from one of its discrete energy states to another. However, many of his peers at the time – including Erwin Schrödinger – strongly objected to their existence, instead postulating instead that they are not instantaneous.
Researchers didn’t actually observe quantum jumps until 1986 (in individual atoms). Since then, they have seen them in various atomic and solid-state systems and have shown that they are an essential phenomenon in processes such as quantum feedback control, and in particular for detecting and correcting quantum errors caused by decoherence in quantum information systems. Quantum error correction is a key challenge in the development of fully-functioning, real-world quantum computers.
Experiments on an artificial three-energy-level atom
A team led by Michel Devoret and Zlatko Minev has now focused on the “canonical case” of quantum jumps between two levels indirectly monitored by a third. This case, they say, corresponds to the 1986 tests of Bohr’s original idea on quantum jumps.
The researchers did their experiments on a superconducting electrical circuit that behaves like an atom with three energy levels. These are: the ground state; an auxiliary “bright” state that can be connected to the ground state by a transition stimulated by microwave light; and a “dark” state into which the atom can jump.
Using a technique called indirect quantum non-demolition measurement, they shone a microwave light beam at the artificial atom, with a frequency corresponding to the ground-bright transition. Thanks to the energy of the light beam, the atom rapidly bounces between the ground and bright state, emitting a photon each time it jumps from the bright to the ground. If the atom absorbs a higher-energy photon, from another light beam, however, it jumps into the dark state. When the atom is in the bright state, it scatters yet another light beam and when it is in the dark state it does not scatter it.
Observing when a quantum jump has begun
“When the atom is cycling between the ground and bright states, we observe a bright scattered light field,” explains Minev. “But, when the atom is in the dark state, we observe no light.”
“What is new in this work is that we can resolve essentially every single scattered photon with near perfect measurement efficiency.”
This perfect measurement efficiency comes thanks to a scheme proposed by Minev and it allows the researchers to observe when a quantum jump has begun, by identifying the missing flash of light scattered from the bright state.
Latency period
Just before a jump occurs, however, there is always a latency period (of a few microseconds), during which it is possible to acquire a signal that warns of the upcoming jump, say the researchers. This “advance warning signal” consists of a lull in the scattered light as the atom jumps into the dark state.
Minev says that he proposed the experiment inspired by a theoretical prediction by Howard Carmichael of the University of Auckland, a pioneer of quantum trajectory theory and a co-author of this study.
“Our experimental result shows that while quantum jumps are unpredictable and discrete (as Bohr thought) on long timescales, they can be continuous (as Schrodinger suggested) and predictable on a short time scale,” says Devoret.
Quantum jumps are not truly instantaneous and random
The researchers did not stop there: they also managed to control the quantum jump once it had started by applying an electric pulse to the artificial atom. In this way, they intercepted it and sent it back to the ground state. They are only able to do this because the quantum jump is not truly instantaneous and random. Instead, quantum jumps take the same trajectory between the two energy levels every time, so it is possible to predict how to send them back.
According to the Yale team, this is an important point: “while quantum jumps appear discrete and random in the long run, reversing a quantum jump means the evolution of the quantum state possesses, in part, a deterministic and non-random character,” say Devoret and Minev. “The jump always occurs in the same, predictable manner from its random starting point.”
“The findings are in complete agreement with the predictions of modern quantum trajectory theory,” Devoret tells Physics World, “with essentially no adjustable parameters.
“One of the applications for the type of experiment performed in our study is an efficient method for detecting very weak signals buried in quantum noise, and real-time intervention based on the results of this detection.”

The quantum heretics
“The results should also provide new ground for exploring intervention techniques to control quantum systems, such as the early detection of error syndromes in quantum error correction,” adds Minev. An unexpected jump could hint at a mistake in calculations, for instance, and might allow researchers to spot the beginning of the jump and account for the error – or perhaps even reverse it mid-jump.
The researchers, reporting their work in Nature 10.1038/s41586-019-1287-z, say they would now like to generalize their experiment to a situation involving two distant measurements on a common entangled system and see how the two distant jumps correlate. “This would be analogous to dissecting a Bell-inequality violation measurement,” says Devoret.