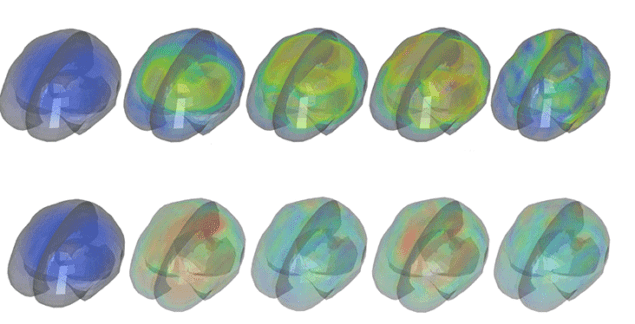
Numerical simulations have revealed how motions of the brain during head impacts can be characterized by just a few low-frequency modes of vibration in brain tissue. The study, led by Kaveh Laksari at the University of Arizona and Mehmet Kurt of the Stevens Institute of Technology in New Jersey, used sensors in the helmets of American football players to record head impacts. The motion of the brain was then recreated in computer simulations. Their research gives new insights into the mechanics responsible for traumatic brain injury (TBI).
The simple dynamics of head impacts have been well understood for decades. As the skull accelerates rapidly during an impact, the brain lags behind because its motion is damped by the various membranes and fluids connecting it to the skull. However, the brain is a remarkably complex and heterogeneous material and its many different parts have varying mechanical properties that respond differently to forces. As a result, established models of brain motion during a head impact are too simple to be able to predict whether a TBI has occurred. This is an important shortcoming because TBI is currently the single largest cause of injury-related deaths in the US.
Experiments on volunteers
Previous attempts to improve our understanding brain motion have involved experiments on volunteers subjected to mild head accelerations while inside a magnetic resonance imaging (MRI) machine. Meanwhile, studies of more violent injury-inducing impacts are done on cadavers. Computer simulations have also provided important insights into brain motion, allowing for predictions of the motions of the brain as it responds to different accelerations.
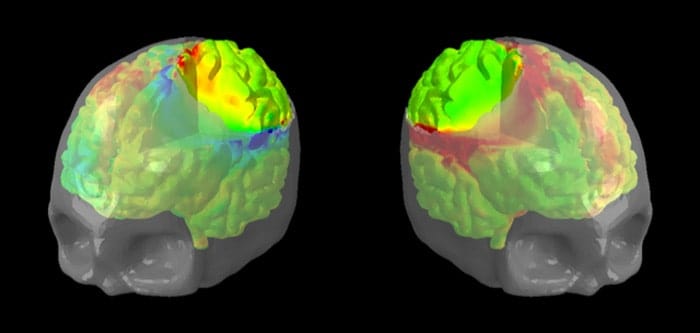
Simulating inner strain that causes brains to bulge
To improve these simulations, the team used a relatively new technique called “dynamic mode decomposition” (DMD) to analyse data from impacts. DMD bears some similarity to Fourier analysis, but calculates a new set of vibrational modes from every set of data gathered. This allows the motion of the brain to be represented using fewer modes than Fourier analysis, which is particularly useful for understanding the damped movements of a bound, heterogeneous material like the brain.
Football helmets
The team gathered their data by attaching motion sensors to the helmets of American football players to measure 187 head impacts that occurred during games and training. They then used the measurements as input to a head impact simulation, using DMD to break down the resulting strain and displacement fields in the simulated brain into a few damped, oscillating modes of vibration. To their surprise, they found that the impact fields could be closely approximated using just a few modes, with frequencies around 40 Hz.
The researchers also found that milder impacts were characterized by a single mode across the entire brain, while stronger impacts required larger numbers of modes. They team believe they have shown for the first time that brain injuries are caused by vibrational modes induced by impacts, rather than the peak acceleration experienced by the head. This could help explain why their is a low correlation between peak acceleration and brain injury in patients. However, the team does emphasize that more clinical studies are required to back up the connection between vibration and injury.
The research is described in Physical Review Letters.