THERYQ is developing a radiotherapy system that uses very high-energy electrons to treat deep-seated tumours, and can also enable FLASH treatments
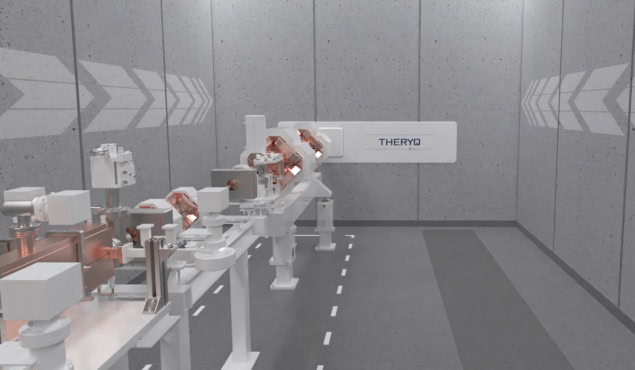
Electron therapy has long played an important role in cancer treatments. Electrons with energies of up to 20 MeV can treat superficial tumours while minimizing delivered dose to underlying tissues; they are also ideal for performing total skin therapy and intraoperative radiotherapy. The limited penetration depth of such low-energy electrons, however, limits the range of tumour sites that they can treat. And as photon-based radiotherapy technology continues to progress, electron therapy has somewhat fallen out of fashion.
That could all be about to change with the introduction of radiation treatments based on very high-energy electrons (VHEEs). Once realised in the clinic, VHEEs – with energies from 50 up to 400 MeV – will deliver highly penetrating, easily steerable, conformal treatment beams with the potential to enable emerging techniques such as FLASH radiotherapy. French medical technology company THERYQ is working to make this opportunity a reality.
Therapeutic electron beams are produced using radio frequency (RF) energy to accelerate electrons within a vacuum cavity. An accelerator of a just over 1 m in length can boost electrons to energies of about 25 MeV – corresponding to a tissue penetration depth of a few centimetres. It’s possible to create higher energy beams by simply daisy chaining additional vacuum chambers. But such systems soon become too large and impractical for clinical use.
THERYQ is focusing on a totally different approach to generating VHEE beams. “In an ideal case, these accelerators allow you to reach energy transfers of around 100 MeV/m,” explains THERYQ’s Sébastien Curtoni. “The challenge is to create a system that’s as compact as possible, closer to the footprint and cost of current radiotherapy machines.”
Working in collaboration with CERN, THERYQ is aiming to modify CERN’s Compact Linear Collider technology for clinical applications. “We are adapting the CERN technology, which was initially produced for particle physics experiments, to radiotherapy,” says Curtoni. “There are definitely things in this design that are very useful for us and other things that are difficult. At the moment, this is still in the design and conception phase; we are not there yet.”
VHEE advantages
The higher energy of VHEE beams provides sufficient penetration to treat deep tumours, with the dose peak region extending up to 20–30 cm in depth for parallel (non-divergent) beams using energy levels of 100–150 MeV (for field sizes of 10 x 10 cm or above). And in contrast to low-energy electrons, which have significant lateral spread, VHEE beams have extremely narrow penumbra with sharp beam edges that help to create highly conformal dose distributions.
“Electrons are extremely light particles and propagate through matter in very straight lines at very high energies,” Curtoni explains. “If you control the initial direction of the beam, you know that the patient will receive a very steep and well defined dose distribution and that, even for depths above 20 cm, the beam will remain sharp and not spread laterally.”
Electrons are also relatively insensitive to tissue inhomogeneities, such as those encountered as the treatment beam passes through different layers of muscle, bone, fat or air. “VHEEs have greater robustness against density variations and anatomical changes,” adds THERYQ’s Costanza Panaino. “This is a big advantage for treatments in locations where there is movement, such as the lung and pelvic areas.”
It’s also possible to manipulate VHEEs via electromagnetic scanning. Electrons have a charge-to-mass ratio roughly 1800 times higher than that of protons, meaning that they can be steered with a much weaker magnetic field than required for protons. “As a result, the technology that you are building has a smaller footprint and the possibility costing less,” Panaino explains. “This is extremely important because the cost of building a proton therapy facility is prohibitive for some countries.”
Enabling FLASH
In addition to expanding the range of clinical indications that can be treated with electrons, VHEE beams can also provide a tool to enable the emerging – and potentially game changing – technique known as FLASH radiotherapy. By delivering therapeutic radiation at ultrahigh dose rates (higher than 100 Gy/s), FLASH vastly reduces normal tissue toxicity while maintaining anti-tumour activity, potentially minimizing harmful side-effects.
The recent interest in the FLASH effect began back in 2014 with the report of a differential response between normal and tumour tissue in mice exposed to high dose-rate, low-energy electrons. Since then, most preclinical FLASH studies have used electron beams, as did the first patient treatment in 2019 – a skin cancer treatment at Lausanne University Hospital (CHUV) in Switzerland, performed with the Oriatron eRT6 prototype from PMB-Alcen, the French company from which THERYQ originated.
FLASH radiotherapy is currently being used in clinical trials with proton beams, as well as with low-energy electrons, where it remains intrinsically limited to superficial treatments. Treating deep-seated tumours with FLASH requires more highly penetrating beams. And while the most obvious option would be to use photons, it’s extremely difficult to produce an X-ray beam with a high enough dose rate to induce the FLASH effect without excessive heat generation destroying the conversion target.
“It’s easier to produce a high dose-rate electron beam for FLASH than trying to [perform FLASH] with X-rays, as you use the electron beam directly to treat the patient,” Curtoni explains. “The possibility to treat deep-seated tumours with high-energy electron beams compensates for the fact that you can’t use X-rays.”
Panaino points out that in addition to high dose rates, FLASH radiotherapy also relies on various interdependent parameters. “Ideally, to induce the FLASH effect, the beam should be pulsed at a frequency of about 100 Hz, the dose-per-pulse should be 1 Gy or above, and the dose rate within the pulse should be higher than 106 Gy/s,” she explains.
Into the clinic
THERYQ is using its VHEE expertise to develop a clinical FLASH radiotherapy system called FLASHDEEP, which will use electrons at energies of 100 to 200 MeV to treat tumours at depths of up to 20 cm. The first FLASHDEEP systems will be installed at CHUV (which is part of a consortium with CERN and THERYQ) and at the Gustave Roussy cancer centre in France.
“We are trying to introduce FLASH into the clinic, so we have a prototype FLASHKNiFE machine that allows us to perform low-energy, 6 and 9 MeV, electron therapy,” says Charlotte Robert, head of the medical physics department research group at Gustave Roussy. “The first clinical trials using low-energy electrons are all on skin tumours, aiming to show that we can safely decrease the number of treatment sessions.”
While these initial studies are limited to skin lesions, clinical implementation of the FLASHDEEP system will extend the benefits of FLASH to many more tumour sites. Robert predicts that VHEE-based FLASH will prove most valuable for treating radioresistant cancers that cannot currently be cured. The rationale is that FLASH’s ability to spare normal tissue will allow delivery of higher target doses without increasing toxicity.
“You will not use this technology for diseases that can already be cured, at least initially,” she explains. “The first clinical trial, I’m quite sure, will be either glioblastoma or pancreatic cancers that are not effectively controlled today. If we can show that VHEE FLASH can spare normal tissue more than conventional radiotherapy can, we hope this will have a positive impact on lesion response.”
“There are a lot of technological challenges around this technology and we are trying to tackle them all,” Curtoni concludes. “The ultimate goal is to produce a VHEE accelerator with a very compact beamline that makes this technology and FLASH a reality for a clinical environment.”