Anna Demming discovers why the vacuum-based technique of atomic-layer deposition – a variant of chemical-vapour deposition – holds so much promise for energy and environmental applications
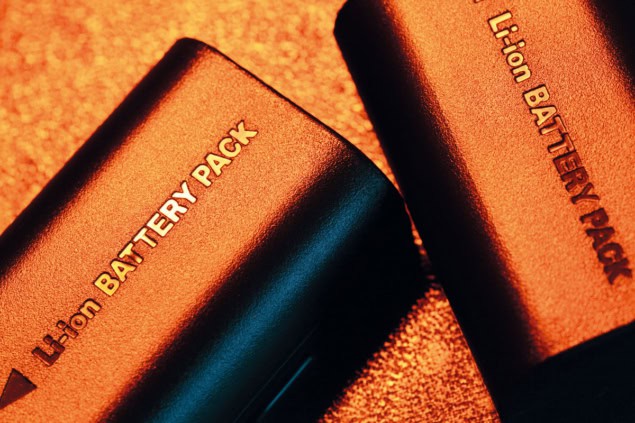
Tools are useful when they meet all the demands of a particular objective, and invaluable when they continue to meet requirements that are ever evolving over time. Atomic-layer deposition (ALD) was already a useful tool for thin films in the 1980s, although commercial applications were then limited to electroluminescent displays. By the 1990s, use of ALD was making inroads into the microelectronics industry, but it was not until the end of that decade that the great match between the fabrication requirements in nanotechnology and the precision and control the technique can achieve became apparent.
Interest in the technique was confirmed in the early 2000s, when the American Vacuum Society began an international conference series on ALD. Since then, more than 1200 research papers, 80 reviews and two books have been published on ALD in nanotechnology. Some of the latest research using this technique to make devices for energy and environmental applications have appeared in a recent focus collection of the journal Nanotechnology from IOP Publishing, which also publishes Physics World.
ALD is a variant of the widely used technique of chemical-vapour deposition (CVD), in which a thin film is grown on a substrate by exposing it to one or more volatile gases – known as precursors – that react or decompose on the substrate to produce a required structure. The big difference with ALD is that the precursors are never present at the same time. Instead, ALD involves exposing the surface of a material to atoms of the chemicals to be deposited in separate stages and then clearing the excess between each stage.
In each of these stages, the precursor molecules react with the surface in a self-limiting way, which means that the reaction stops when all of the reactive sites on the surface are consumed. As a result, the amount of material that can be deposited on the surface after a single exposure to all of the precursors (a so-called ALD cycle) is governed by the nature of the precursor–surface interaction. By varying the number of cycles, researchers can therefore grow materials uniformly and with high precision on arbitrarily complex and large substrates.
In a paper published in the late 1990s exploring how ALD might be useful in nanofabrication (Nanotechnology 10 19), Mikko Ritala and Markku Leskelä of the University of Helsinki list the merits this tweak introduces. These include “accurate and simple film-thickness control, sharp interfaces, uniformity over large areas, excellent conformality, good reproducibility, multilayer processing capability and high film qualities at relatively low temperatures”. These attributes have proved particularly valuable for attempts to improve the efficiency and scalability of alternative-energy technologies.
Coating electrolytes on 3D structures
Many of the processes key to energy harvesting and storage devices are improved by the increased surface area that 3D structures bring. For lithium-ion batteries, a higher surface area means higher energy and power densities, as well as space to accommodate the addition and removal of lithium ions, which can introduce mechanical strain during use. Researchers studying lithium-ion batteries are therefore increasingly looking towards solid state as opposed to liquid electrolytes to avoid risks of leakage and corrosion, with sophisticated coating techniques to add electrolyte and counter-electrode layers to complex 3D nanostructures therefore being in high demand.
ALD not only provides pinhole-free coatings of solid-state electrolytes on 3D electrode structures, but it also lets researchers tailor the coating thickness, which can significantly improve performance. As Tsun-Kong Sham and Xueliang Sun and colleagues at the University of Western Ontario in Canada point out (Nanotechnology 25 504007), “It is expected that the lithium-phosphate thin films prepared by ALD can find potential applications as solid-state electrolytes for 3D all-solid-state micro lithium-ion batteries, which, as an emerging area, deserves more extensive investigation in the coming future.”
Supercapacitors are a particularly attractive energy resource in nanoelectronics, where space is at a premium. As with research on lithium-ion batteries, the advantages of 3D structures with solid-state electrolytes have been noted. Writing in the Nanotechnology focus collection (26 064002), Giuseppe Fiorentino, Frans Tichelaar and their colleagues at Delft University of Technology in the Netherlands report on supercapacitors made from carbon-nanotube bundles as high-aspect-ratio electrodes that can improve the capacitance by a factor of five. The electrode is coated with aluminium-oxide as the electrolyte, followed by titanium-nitride as the counter electrode. As Fiorentino’s team points out, the work is not only “the first known example of large-scale manufacturable nanostructured capacitors”, but also provides useful insights for coating such high-aspect-ratio nanostructures.
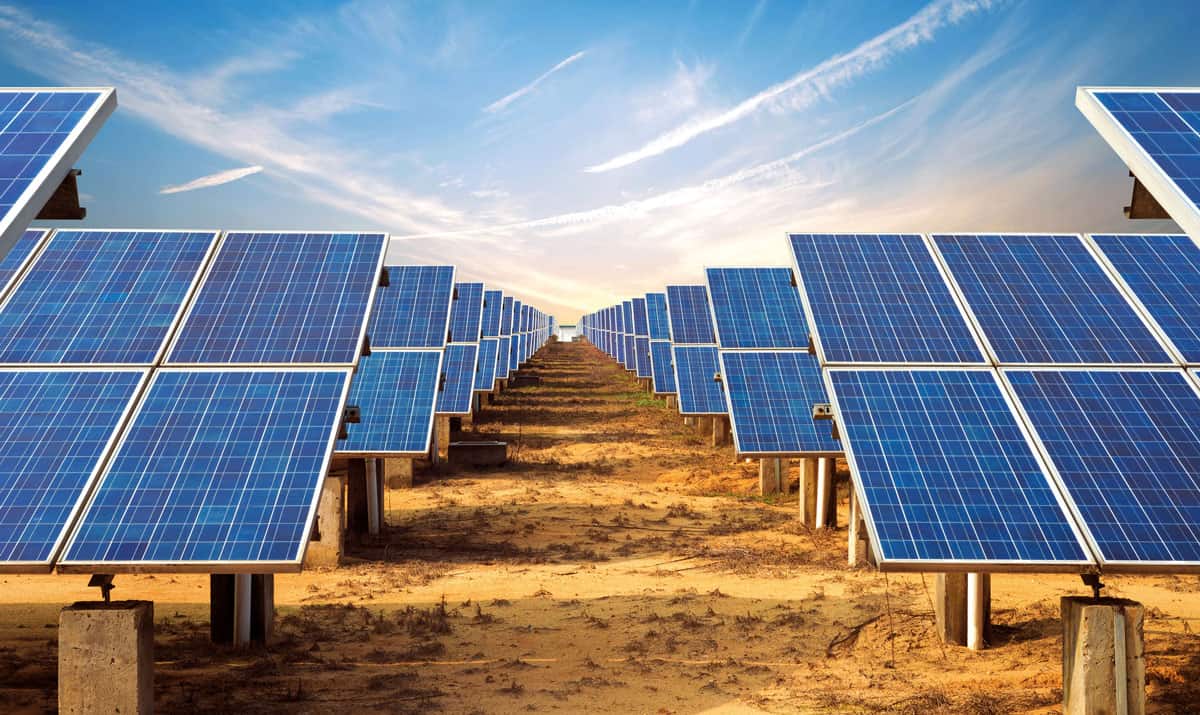
Electrochemical cell structures can also take on elaborate hierarchical forms, as demonstrated by Xudong Wang and colleagues at the University of Wisconsin-Madison and the Forest Products Laboratory in the US. They have combined ALD and cellulose nanofibres to produce extensively branched structures for photo-electrochemical water splitting. Such a 3D titanium-dioxide fibre-nanorod heterostructure offers, they say, “a new route for a cellulose-based nanomanufacturing technique, which can be used for large-area, low-cost and green fabrication of nanomaterials as well as their utilizations for efficient solar-energy harvesting and conversion”.
As for solar-energy harvesting, ALD is already a well-entrenched technique in the field, and has been used to coat the inverse opals and other 3D structures often employed to reduce the distances that charge carriers must travel to reach electrodes. In the focus collection of Nanotechnology (26 064001), Alfred Iing Yoong Tok and colleagues at Nanyang Technological University in Singapore and the University of New South Wales in Australia review the application of ALD in solar-power technologies, focusing on its use for surface passivation, surface sensitization and band-structure engineering.
The potential for lateral confinement
As well as providing an excellent tool for coatings, ALD offers great potential for controlled lateral confinement. This aspect is the subject of detailed scrutiny in the catalysis work of Marcel Verheijen at Philips Innovation Services and Eindhoven University of Technology in the Netherlands. Coating thickness is still key for catalysis performance, as demonstrated by Ai-Dong Li and colleagues at Nanjing University in China. However, as the work by Verheijen and his colleagues in the group of Wilhelmus Kessels at the university identifies, ALD can also help with size matters. In a study of four ALD processes for the preparation of nanoparticle catalysts made from platinum and palladium, they identify the potential for size control, as well its dependence on process conditions (Nanotechnology 27 034001).
It is the ability to accommodate innovation that makes atomic-layer deposition so invaluable
It is perhaps the ability to accommodate innovation that makes ALD so invaluable. The use of plasmonic metamaterial absorbers has only really gained notice in the past few years, and ALD is already proving invaluable for work exploring the essential mechanisms in these systems, too. Xin Chen and colleagues at the Shanghai Institute for Technical Physics, for example, exploit ALD control over dielectric layers in order to distinguish between different plasmon modes. “We have demonstrated inversed plasmonic metamaterial absorber architectures with a tuneable ALD spacer layer, and thus identified the contributions of the gap plasmon and the interference-enhanced local surface plasmon resonance to the superior absorption in a step-by-step manner,” they say.
It is typical of science to break down problems into manageable pieces that can be tackled step by step. By breaking down deposition to an atomic level with step-by-step, self-limiting stages, ALD mirrors this approach and in so doing seems to provide a multipurpose tool for a diverse range of applications.
- The Nanotechnology focus collection on energy and environmental applications of atomic-layer deposition is available at this link.