
Solar cells based on wide-band-gap semiconductors work better under water than the narrow-band-gap ones used in conventional silicon photovoltaic devices. This finding by researchers at New York University (NYU), US, could aid the development of more efficient solar cells to power autonomous submersible vehicles and sensors.
Underwater vehicles cannot operate for long periods of time due to the lack of durable power sources. At present, they typically rely on batteries, connections to shore-based grid power, or power derived from solar cells situated above water – for example on a surface ship.
Onboard solar panels could be an attractive alternative for vehicles that need long-term power far from shore or support vessels, but conventional solar cells based on silicon or amorphous silicon are far from ideal for underwater operation. Thanks to their narrow band gaps of around 1.1 and 1.8 eV respectively, cells of this type absorb a large amount of red and infrared light. Unfortunately, water is also very good at absorbing these wavelengths, even at shallow depths, explains study lead author Jason A Röhr.
Blue and yellow light (400–600 nm), in contrast, is less easily absorbed by water, which means that it can penetrate further below the surface. To Röhr and his colleagues in André Taylor’s Transformative Materials and Devices Lab at NYU’s Tandon School of Engineering, this suggested that semiconductors with wider band gaps could be a better “fit” for supplying energy underwater, he says.
Detailed-balance model
To investigate these types of semiconductors further, the team used a detailed-balance model to measure the efficiency limits of different light-absorbing materials in various oceans and lakes around the world. These included parts of the Atlantic and Pacific oceans that are relatively clear and absorb light well, and a lake in Finland, which is more turbid and has poorer light absorption characteristics.
The model calculates the power-conversion efficiency of an underwater solar cell, which is given by the ratio of the power density output, pout, to the power density input from the solar spectrum, pin. It then measures the output power from the maximum power point on the solar cell current density-voltage (J-V) curve, pout = JmaxVmax, and measures pin by integrating the photon flux density – that is, the number of photons hitting the cell’s surface – over time. By combining a given absorption spectrum together with known values for the band-gap energy of a semiconductor, the depth below sea level, and water temperature, the researchers then obtained the detailed-balance efficiency limit of a semiconductor material in different locations.
For their input data, the researchers sifted through more than 400 published articles to unearth light-absorption spectra that not only covered the relevant spectral region, but was also representative of many areas on Earth. “We wanted to make sure that we didn’t just consider the clearest waters,” Röhr explains.
The calculations, which are detailed in Joule, reveal that at a depth of two metres, solar-cell absorbers would function best with a band gap of 1.8 eV, while at 50 metres a band gap of 2.4 eV was optimal. The devices’ balance efficiency ranged from around 55% in shallow waters to more than 65% at greater depths, while generating power greater than 5 mW/cm2. These efficiencies increase in cold water, and the researchers found that both balance efficiency and power output were independent of geographic location. This is good news because it means that the solar cells could be tailored to specific operating depths rather than locations.
The best underwater semiconductors
So which types of semiconductors might best fit these requirements? According to Röhr, good candidates include solar cells made from organic materials, which are lightweight, cheap to make and work well under low light conditions. Poly(3-hex- ylthiophene-2,5-diyl) (P3HT), for example, has a band gap of around 2.1 eV, can easily be produced in large quantities, and could be perfect for operation in shallow waters. Materials such as rubrene (2.2 eV band gap) and pentacene (2.2 eV) would work well at large depths, as would poly(p-phenylene vinylene) derivatives (2.3–2.4 eV).
Alternatively, alloys made with elements from groups III and V of the periodic table, such as the ternary and quaternary cadmium zinc telluride (CZT), copper zinc antimony sulphide (CZAS), AlGaAs, InGaP and GaAsP could also be good because they have tuneable band gaps, and so could be tailored to more efficiently absorb light at various depths.
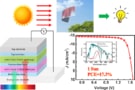
Organic solar cells break new efficiency record
While the composition of underwater sun-harvesting materials would be different to conventional solar cells, their general design would not need to change all that much, says Röhr. Obviously, they would need to be waterproof and stable for long periods in marine environments, but researchers have already shown that silicon solar panels can be encapsulated and remain submerged underwater for months without suffering any significant loss in power conversion. Similar encapsulation techniques might thus be employed to stabilize solar cells made from wide-band-gap semiconductors.
“Alternatively, we recently demonstrated that organic solar cells can be made resilient to water by selectively removing the electron acceptor from the top surface of the material,” Röhr tells Physics World. “However, we still need to show that this type of cell can be made more efficient than traditional silicon-based cells.”
This, he concedes, will be no easy task, as silicon’s cost and efficiency are both so competitive. However, he adds that new materials are constantly being developed, and he hopes that these results will inspire other research groups to look for new materials for underwater cells, too.