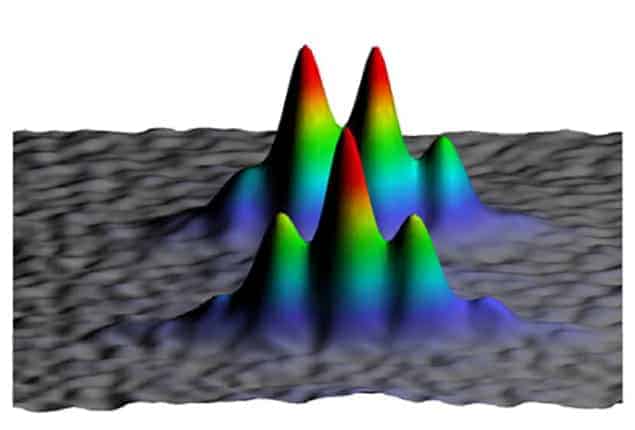
Physicists have used interferometry to detect the minute tidal forces acting on individual atoms exposed to a local gravitational field. This allowed them to measure the curvature of space–time on a very small scale and argue that their observations are perhaps “the first of gravity in a quantum-mechanical system”. The team adds that its sensitive apparatus might also improve prospecting for oil and minerals.
Tidal forces arise due to the finite size of gravitating objects. When one body is attracted to another it will experience a larger attractive force on the side nearest the second body than it will on the far side. In the case of the Earth, the oceans nearest the Moon are attracted slightly more to our celestial neighbour than are the oceans on the far side of the planet, causing the former to bulge slightly and create a high tide.
In the latest work, Mark Kasevich at Stanford University in the US and colleagues instead measure the minuscule tidal forces created when single atoms are allowed to fall in the presence of a nearby massive object. They did so to study the effect of space–time curvature on the atoms’ wave functions – tidal forces being directly related to this curvature – with the idea that gravity might conceivably destroy quantum coherence.
Wave–particle duality
The team used an atom interferometer, a device that exploits the principle of wave–particle duality to enable very precise measurements of acceleration. Like an optical interferometer, it involves splitting a particle’s wave function, sending the two halves along different paths and then recombining the separate waves to establish whether or not the waves’ relative phase has changed along the way.
The Stanford group propelled ultracold rubidium atoms up a 10 m-high tube and then fired a precise series of laser pulses at the atoms as they fell back down to Earth. These pulses acted as the equivalent of beam splitters, mirrors and other components in an optical interferometer.
Wave packets of individual atoms were divided such that the two parts travelled inside different interferometers placed 30 cm apart. One of the interferometers was created close to an 84 kg lead mass, and it was the roughly 10 cm-wide partial wave packet that travelled through this device that was monitored for evidence of tidal forces. The other interferometer – beyond the gravitational range of the lead – was instead used as a reference to eliminate the effect of Earth’s gravity and instrumental vibrations.
We were able to establish the fingerprint for tidal forces
Mark Kasevich, Stanford University
By varying the distance between the arms in the first interferometer, the researchers found that the resulting differences in relative phase yielded a quadratic dependence in agreement with the calculated space-time curvature created by the lead mass. “We were able to establish the fingerprint for tidal forces,” says Kasevich.
This is not the first time that atom interferometers have been used to measure space–time curvature. In 2015, Guglielmo Tino of the University of Florence in Italy and colleagues demonstrated the presence of curvature by comparing the accelerations of atoms within three separate clouds placed in the gravitational field of a set of tungsten-alloy masses. But Kasevich says that atoms in different clouds do not exist in a single coherent quantum state. Only by measuring the tidal force across individual wave packets, he says, would it be possible to observe gravity’s destruction of quantum coherence – should that take place in nature.
Coherence preserved
Kasevich says that the results obtained in the current work are “completely consistent” with the preservation of quantum coherence. As to what level of experimental sensitivity might be needed to observe any decoherence, he says that current theory provides little guidance. Most physicists working on theories of quantum gravity, he adds, are interested in the “strong-field limit” characteristic of black holes and other massive objects, in contrast to the very small fields that generate atomic tidal forces. Nevertheless, he believes it is important to make the measurements. “I am an experimental physicist,” he says. “My job is to push physical systems into new domains.”
According to Kasevich, his group’s work is also important technically since it shows how to carry out quantum measurements on the centimetre, as opposed to millimetre, scale. As for applications, he says the group’s apparatus might potentially improve gravity-based searches for oil and other resources. To identify substances with particular densities under the Earth’s surface, engineers generally employ gravimeters that measure the variation in gravitational acceleration from one point to another. But, as Kasevich explains, the equivalence principle dictates that if such devices are used on board helicopters, for example, it then becomes difficult to distinguish between vibrational and gravitational accelerations. This, he says, is not a problem when measuring space–time curvature.
Hard work needed
Kasevich acknowledges that there “is still a lot of work” needed to make a practical version of their instrument – one that “is not a 10 m-high tower located in the basement of a building”. In particular, he says, lasers must have “reasonable power levels and good control over polarization”. But he believes that these challenges can be overcome, arguing that money from UK, European and US schemes in quantum technology can “make these things come to fruition”.
Tino praises the California team, noting the importance of being able to split wave packets over longer distances than previously. “This is a further demonstration of the potential of atom interferometry for the investigation of gravity in yet unexplored systems,” he says. The work is described in Physical Review Letters.