An international team of astronomers has used an unusual double pulsar to provide the strongest confirmation yet of Einstein’s general relativity -- the theory that physicists believe best explains gravity.
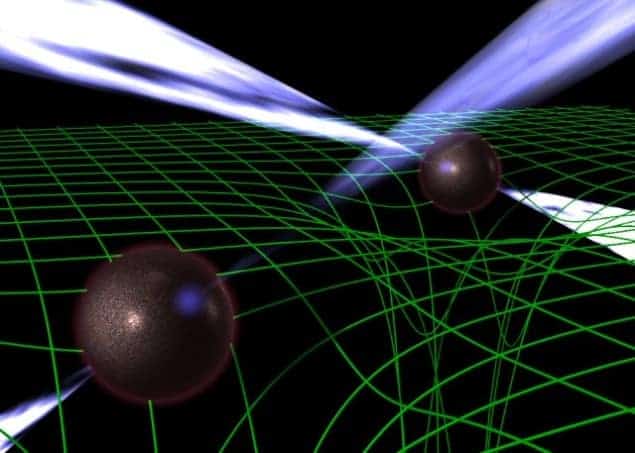
Michael Kramer at Jodrell Bank Observatory and colleagues carried out four separate tests on the pair of rotating neutron stars, verifying general relativity to an astonishing accuracy of 99.5% (Science 313 1556). The team now hopes to improve the precision so that they can eventually probe the internal structure of these superdense stars and perhaps even see the first hints of quantum gravity.
The double pulsar PSR J0737-3039A/B, which was discovered by the Jodrell Bank team in 2003, lies some 2000 light-years away from Earth. It consists of two compact neutron stars, each a mere 20 km across yet weighing more than the Sun and separated by only a million kilometres. Given the tiny size, high mass density and very short orbital period of just 2.4 hours, the double-pulsar system has a gravitational potential 100,000 times that of our Sun — higher than anything else in the universe, apart from black holes
Relativistic effects in this system are therefore much more pronounced and space-time is far more curved than under normal conditions that exist in our solar system. This makes the double pulsar an excellent “laboratory” for testing general relativity, particularly because both stars send out regular beams of radio waves, which can be captured by large telescopes and used to probe the curved space-time around such a system. Such measurements can reveal whether general relativity applies only in the weak-field conditions of our own solar system and if there are deviations in places where gravity is very strong.
Using the Lovell Telescope at Jodrell Bank — as well as the Parkes Radio Telescope in Australia and the Robert C Byrd Green Bank Telescope in West Virginia, USA — the team measured five mathematical parameters that describe relativistic effects as corrections to the simple Keplerian motion of stars. One is the rate at which the pulsars are slowing down and spiralling in towards each other as they lose energy by emitting gravitational waves. Another parameter is the rate at which pulses from one star are being slowed by the other star’s gravitational pull and by the curved space-time around them.
The team also measured the mass ratio of the two stars to be 1.07. This number is important because it can be used with the measured value of one of the five parameters to determine the individual masses of the two pulsars. These masses can then be plugged into the equations of general relativity to calculate theoretical values for the other four parameters, which can then compared be with what their instruments actually measure to see how accurate the theory is.
The conclusion from these four independent tests of GR is clear — the pulsars are behaving as predicted, to an unerring accuracy of 99.5%. Intriguingly, the measurements also suggest the second pulsar was formed from a star of probably less than two solar masses. This is much lower than deemed necessary for supernova explosions, raising new questions about how stars evolve.
Kramer now wants to improve the precision of his measurements still further. “Eventually, we know general relativity should fail, as it does not describe nature on small scales,” he says. Such more precise measurements will put tighter limits on alternative theories of gravity and could eventually show new pulsar interactions, casting light on their superdense internal structure.