Researchers have used the ultrafast X-ray pulses from a free-electron laser to image a nanoscale object in just a femtosecond. The technique, which is a new form of X-ray holography, has been pioneered by Henry Chapman from Lawrence Livermore National Laboratory and colleagues in the US, Sweden and Germany. Being able to study materials so fast brings us one step closer to the holy grail of observing, at the same time, how all the atoms in a molecule move (Nature 448 676).
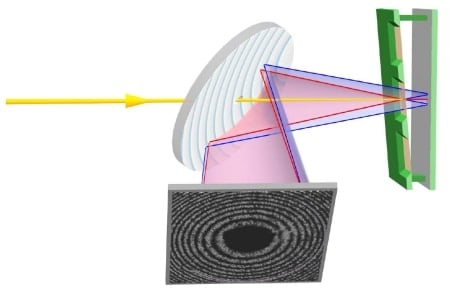
In their technique, Chapman and co-workers start by firing a coherent pulse of light from the X-ray free-electron laser at the DESY lab in Hamburg through a small hole in a “detector” mirror. This pulse then encounters a thin, translucent membrane that has been covered with a sample material – in this case 140-nm-diameter polystyrene balls – that lies just in front of a second, “backing” mirror (see Quick as a flash).
If the pulse hits one of the balls, it strips the polystyrene chains of their electrons, causing the material to explode under the repulsion of the remaining positive charge. The X-rays then scatter off the ball before travelling to the backing mirror, which reflects it.
Because the pulse has a finite width, however, not all of the X-rays interact with the ball – some of them bypass it and continue in their original direction before bouncing off the backing mirror. These X-rays can then scatter off the ball, albeit a fraction of a second after the initial scattering event, by which time the ball has got bigger as a result of the explosion.
Both parts of the now-scattered pulse – known as the “reference” and “object” beams – then travel back to the detector mirror, which reflects them onto a digital camera whereupon they interfere with each other to produce a “holographic” pattern. The researchers then analyze this pattern to reveal the structure of the sample’s material and how it evolved during the explosion.
Although other X-ray holography techniques have been used as far back as the 1970s, Chapman and co-workers’ technique is much faster, having a temporal resolution of just one femtosecond. This is the timescale of atomic motion, meaning that different samples could be imaged to see the stages in, for example, chemical reactions. “This is certainly the fastest hologram ever recorded,” Chapman told physicsworld.com.
Since a relatively long wavelength of 32.5 nm was used in this experiment, the spatial resolution of the technique currently stands at 50 nm, but Chapman explains that with future, shorter-wavelength free-electron lasers it should be possible to resolve features as small as 1 nm.
The researchers say they were inspired to make holographic patterns in this way by Isaac Newton, who noticed that sunlight produced “strange and surprising” light and dark bands on a screen after he had bounced it off a mirror speckled with dust particles.