Devices that exploit the spin -- as well as the charge -- of electrons could be one step closer to commercial reality after researchers in the US claim to have transported spin-polarized electrons in silicon for the first time. In their tiny device, electrons are made to flow from a ferromagnetic alloy into a piece of silicon, where they travel for a distance of about 10 µm without losing their polarization. The team has also been able to rotate the electron spins as they travel through the silicon and finally extract the electrons and measure their polarization (Nature 447 295).
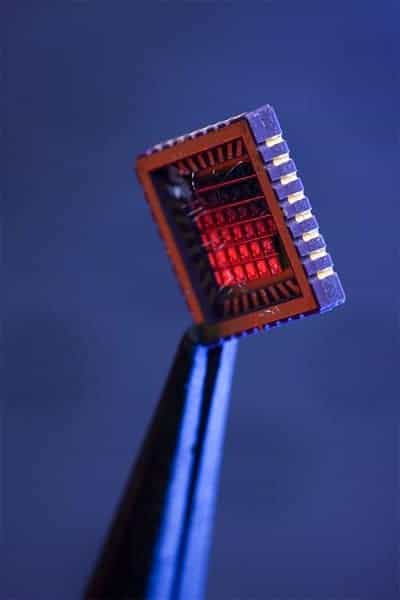
Spintronic devices are electronic circuits that could use both the charge and spin of electrons to transmit, store and process information. In principle, such devices could boost the processing power of conventional computers or even be used in quantum computers.
Silicon should be an ideal material for spintronic devices because the electrons are expected to travel far further than they can in metals without losing their spin polarization. In addition, silicon is the material of choice in the electronics industry, so silicon-based spintronics should be compatible with today’s commercial chip-making processes.
The problem is that it has always been impossible to get the spin-polarized electrons into the silicon in the first place. Spin-polarized electrons are usually found in ferromagnetic materials such as iron, where the spins of most conduction electrons point in the direction of magnetization. If a layer of ferromagnetic metal is bonded to a piece of silicon, the electrons can be made to flow from the magnet into silicon by applying a voltage. Unfortunately, the electrons lose their polarization as they cross the interface between the two materials thanks to an “impedance mismatch” between metal and semiconductor.
This problem has been circumvented in other semiconductor materials such as gallium arsenide by allowing the spin-polarized electrons to “tunnel” across the interface, thus avoiding the impedance mismatch. This however, requires a very thin and abrupt interface between the metal and the semiconductor, which cannot be achieved when growing layers of ferromagnetic metal on silicon.
Now, Ian Appelbaum and Biqin Huang of the University of Delaware and Douwe Monsma at Cambridge NanoTech in Massachusetts have found a new way around the impedance-mismatch problem by sending higher energy “hot” electrons across the metal semiconductor interface. Instead of behaving like an electrical current driven by an applied voltage – which is affected by impedance – these electrons act more like bullets being fired across the interface and are immune to impedance effects. As a result, these “ballistic” electrons travel into the silicon without losing their polarization.
The researchers first created the hot electrons in a tunnel junction that is attached to a 5 nm layer of a ferromagnetic cobalt-iron alloy. The electrons were then injected into the ferromagnet, where they became spin polarized before entering the silicon. After crossing the silicon, the electrons then entered a second ferromagnetic layer, which can measure the spin polarization of the electrons after they have crossed the silicon.
By applying a magnetic field to the device, Appelbaum and colleagues were able to rotate the direction of spin-polarization as the electrons travelled through the silicon. The degree of precession could be altered by varying the magnetic field or by changing the velocity of the electrons by applying an electric field. By observing this precession, the researchers were able to confirm that the electrons did indeed retain their spin polarization while in the silicon.
The measurements had to be done at the very low temperature of 85 K to minimize leakage currents in the device. While such temperatures are hardly practical, Appelbaum told Physics Web that the device has provided important insights into the materials science of spin transport in silicon.