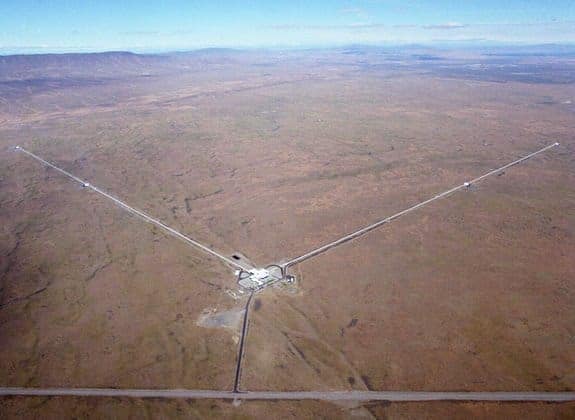
Astrophysicists looking for gravitational waves should set their sights on mountainous neutron stars, according to a new numerical study by researchers in the US. The study also suggests that the crust of a neutron star is 10 billion times stronger than steel — and the researchers say that understanding why could lead to the development of stronger materials here on Earth
General relativity predicts that tiny ripples in the fabric of space time known as gravitational waves are created whenever a massive body is accelerated. Researchers look to space for gravitational waves because only the most massive bodies in the universe can create waves with measurable amplitudes. However, these ripples are still so faint that none has been detected to date.
One such object is a neutron star, the extremely dense core of a collapsed star in which most of the protons and neutrons inside normal matter have been forced together to form neutrons. Any irregularities — or ‘mountains’ — on the surface of a rotating neutron star will cause the star to radiate gravitational waves because the degree of curvature of space-time by a mountain changes slightly depending on whether the mountain is moving towards or away from an observer.
Are detectors sensitive enough?
Physicists are currently looking for gravitational waves using a number of enormous interferometers located around the world. However, researchers have been unsure as to whether current interferometers are sensitive enough to detect gravitational waves from neutron stars because of uncertainties in the size of their mountains.
Now Charles Horowitz of Indiana University and Kai Kadau of Los Alamos National Laboratory in New Mexico have shown that mountainous neutron stars should produce gravitational waves that are strong enough to be seen by existing experiments.
“Our results show that ongoing searches for gravitational waves may have a better chance of success than previously thought,” says Horowitz. “The detection of gravitational waves would confirm a fundamentally new prediction of Einstein’s general relativity and would almost certainly be considered for a Nobel Prize. Detecting gravitational waves from neutron stars would also tell us more about the behaviour of matter at extreme densities.”
Horowitz and Kadau have calculated precisely how high such mountains can be before they collapse under the extreme gravity of a neutron star. They used a computer program to simulate the long-range electrical forces between ions within a neutron star’s crust. These Coulomb interactions hold the crust together and therefore keep mountains in place. The ions themselves are formed from some of the protons that remain from the original star as well as some neutrons.
The physicists modelled how a neutron star’s crust deforms under shear stress. To do this they simulated multiple layers of ions interacting with thousands of their neighbours via the Coulomb force and then let the system evolve as they moved the uppermost and lowermost layers relative to one another. They found that when considering the crust as a pure single crystal the system had a breaking strain of between 0.1 and 0.15, in other words that the shape of the crust can be deformed by up to 10-15% before it breaks.
Strong enough for LIGO
They also showed that impurities, defects and grain boundaries do not reduce this breaking strain to below 0.1. Calculating the height of mountains and therefore the ellipticity that can be supported by such a breaking strain in a typical neutron star, the researchers concluded that such a star will generate gravitational waves that are strong enough to be detectable by LIGO, two pairs of interferometers located in the US.
Horowitz says that the simulation of crust breaking will also improve understanding of “magnetar giant flares”, extremely energetic gamma–ray bursts produced by neutron stars. Researchers believe that these flares result from the energy liberated when a neutron star’s magnetic field lines break the crust and then reconnect when the crust moves. “Now that we have a better understanding on how and when the crust breaks, we can improve this starquarke model to make more detailed comparisons with observations,” he adds.
In addition, Horowitz and Kadau claim that their work could lead to the development of stronger engineering materials. They point out that the enormous strength of a neutron star’s crust — some 10 billion times that of steel — is due to the very long-range Coulomb interactions between ions and the huge pressure exerted on the crust. The latter prevents the formation of voids, which might otherwise cause the material to fail. “It may be possible to design strong conventional materials with these two features,” says Horowitz.
The study is published in Physical Review Letters.