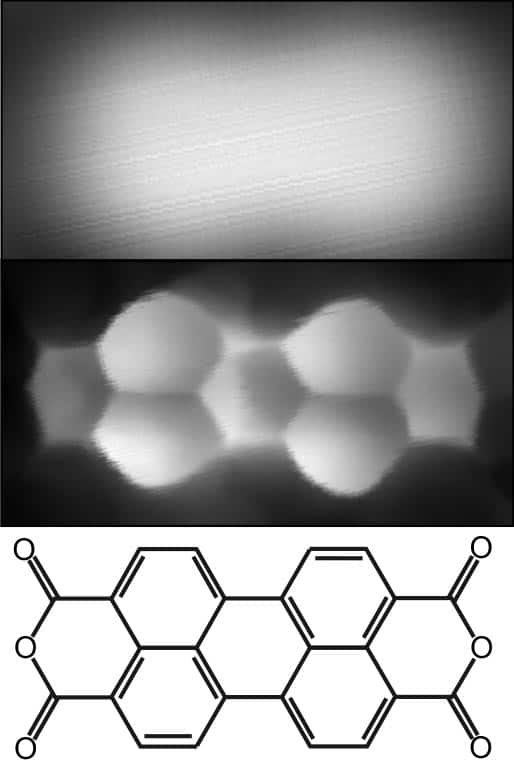
When physicists in Germany discovered a simple way of using a scanning tunnelling microscope (STM) to take images of molecules at the atomic scale for the first time, the technique looked set to make these instruments much more useful for studying molecular structure. But before the method could be used with confidence by the wider scientific community, the researchers involved needed to solve the mystery of why it seemed to work so well.
Now, two years on, the physicists – based at the Forschungszentrum Jülich and at the University of Osnabrück – have shown that the improvement is caused by Pauli repulsion. This is a short-range force that arises from the fact that two or more electrons cannot occupy the same quantum mechanical state.
Hydrogen on the tip
The technique, developed by Stefan Tautz and colleagues, is called scanning-tunnelling hydrogen microscopy (STHM) and involves placing a single molecule of hydrogen right at the metal tip of a conventional STM – something that is easily done by cooling the tip to about 10 K and exposing it to hydrogen gas.
The tip is then brought into contact with the molecule of interest, which is fixed to a metal surface. A small voltage applied between tip and sample causes an electrical current to flow between the two. The tip is raster-scanned across the sample to measure the current as a function of position, creating an image of the molecule.
Pauli repulsion
In conventional STM, the current depends only on the molecule’s valence electrons, which gives little insight into the structure of the molecule. But with hydrogen on the tip, the STM can map the molecule’s total electron density (TED) – which is essentially the molecular structure.
In this latest work, Tautz and colleagues show that if the tip is maintained at a constant height and scanned over the sample, Pauli repulsion between electrons in the hydrogen and electrons in the molecule tends to push the hydrogen into the metal tip. When the tip is over areas of high electron density, the hydrogen is pushed further than when the tip is over regions of low electron density.
When the hydrogen is pushed into the metal tip, conduction electrons are forced away from the tip – another consequence of Pauli repulsion. The result is a drop in the current flowing between the hydrogen and tip.
Seeing intermolecular bonds
In their latest study the researchers looked at the hydrocarbon molecule PTCDA, which forms a herringbone pattern on the surface of gold. As well as imaging individual PTCDA molecules, the team were able to see the very weak bonds between the molecules for the first time.
“The hydrogen molecule is both sensor and signal transducer”, explains Tautz, who adds that the success of the technique also relies on the fact that there is no chemical bonding between the hydrogen and the tip. In other words, the technique could also work using noble gas atoms such as helium and neon.
Now that the physics underlying STHM is understood, Tautz believes that it could be used to identify study and identify complex molecules that have never been seen before. However, one drawback with the technique is that it only works on “flat” molecules that can be attached to a substrate.
Beautifully simple
Despite that problem, STM expert Markus Ternes from the Max Planck Institute for Solid State Research in Stuttgart, Germany, described the findings as “fantastic”, adding that it puts the technique on “solid ground” and will motivate researchers to us STHM as an analytical tool. Ternes, who was not involved in the work, told physicsworld.com that STHM is “beautiful because of its simplicity”.
The work is reported in Physical Review Letters.