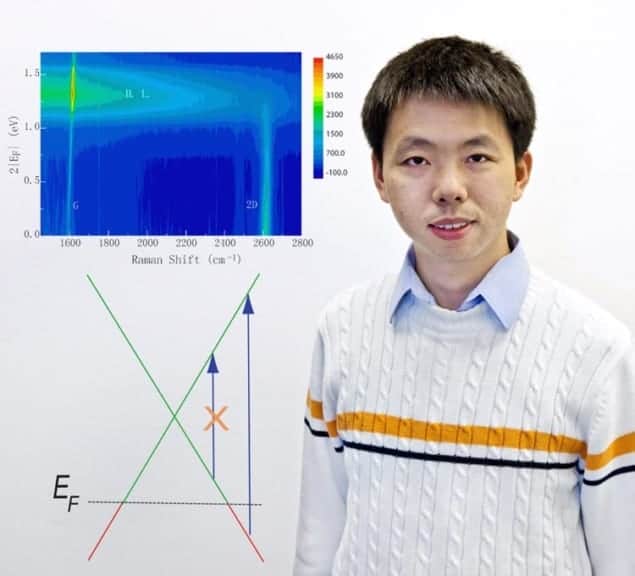
A new technique for manipulating the way light scatters in graphene has been proposed by researchers at the Lawrence Berkeley National Lab and the University of California in the US. They say the breakthrough could help to uncover more information about the structure of the “wonder material” and could lead to the development of nanodevices such as medical sensors.
The technique uses inelastic light scattering spectroscopy, which involves shining laser light onto a material to glean information about the interior from the quantum transitions therein. Photons hitting the sample first excite a set of intermediate electronic states that, in turn, generate phonons (vibrations of the crystal lattice) and so-called energy-shifted photons (those with a higher or lower energy than the incident photons). The intermediate electronic excitations therefore play an important role as quantum pathways in inelastic light scattering.
Feng Wang of Berkeley and colleagues have now observed quantum interference in Raman scattering of light – a form of inelastic light scattering – from graphene for the first time. The researchers have shown that light emission from graphene can be controlled by manipulating the interference pathways thanks to the unique electronic structure of the material. The pathways are electronic excitations that are optically stimulated by the incoming photons and the excitations can only occur if the initial electronic states are filled (by a particle such as an electron) and the final electronic states (which lie above the Fermi level) are empty.
“We were able to control the excitation pathways in graphene by electrostatically doping it – applying voltage to drive down the Fermi energy and eliminate selected states,” said Wang. “An amazing thing about graphene is that its Fermi energy can be shifted by orders of magnitude larger than conventional materials. This is ultimately due to graphene’s two-dimensionality and its unusual electronic bands.”
The team succeeded in lowering graphene’s Fermi energy by coating the material with a special ion gel that contains a strongly conducting liquid in a polymer matrix. The charge in the graphene was adjusted by applying a voltage to the gel.
“By cranking up the voltage we lowered graphene’s Fermi energy, sequentially getting rid of the higher energy electrons,” explained Wang. “Eliminating electrons, from the highest energies down, effectively eliminated the pathways that, when impinged upon by incoming photons, could absorb them and then emit Raman-scattered photons.”
Removing quantum pathways one by one in this manner changes the way that they interfere. These changes can be determined by measuring the Raman-scattered intensity emitted by the sample when it is illuminated with a beam of near-infrared laser light. In Wang and colleagues’ experiment, the intensity actually increases as excitation pathways are removed, contrary to what is expected from classical physics. Wang has dubbed this newly observed phenomenon “a canonical signature of destructive quantum interference”.
Put in simple terms, if lower- and higher-energy pathways interfere destructively in graphene then removing one of them increases the brightness of the light emission.
The team has also discovered something known as “hot electron luminescence”, which was 100 times stronger than the Raman scattering. This light emission comes about from excited electrons jumping up to unfilled bands in samples where graphene’s Fermi energy had been lowered. These hot electrons can only fall back to lower energy bands if they emit a photon of the right frequency.
Being able to control inelastic light scattering in this way could lead to optimized inelastic light scattering in nanomaterials for biological sensors and optoelectronic applications, say the researchers. It could also be a powerful tool for probing novel nanoscale physics in graphene, and indeed other materials. “Likewise the phenomenon of hot electron luminescence could become a valuable research tool too,” added Wang, “particularly for studying ultrafast electron dynamics, one of the chief unusual characteristics of graphene.”
This research is described in a paper in Nature.