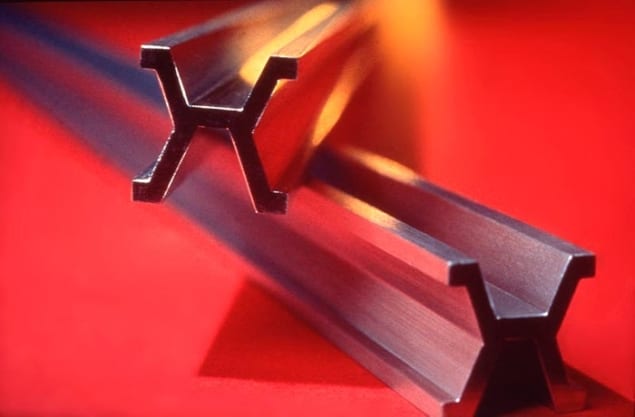
Researchers in Germany have moved one step closer to closing the “quantum metrology triangle”, by fabricating a proof-of-principle circuit that links two quantum electrical devices in series, for the first time. A closed triangle – something scientists have been chasing for more than 20 years – would finally allow standardized units of voltage, current and resistance to be defined solely in terms of fundamental constants of nature.
Metrology – the science of measurement – has evolved as new and more accurate ways of standardizing measurements have been found. For example, the metre is nowadays defined in terms of the speed of light, rather than by the old platinum–iridium prototype, because even though it was held in a controlled environment, the prototype was susceptible to tiny chemical and structural changes over long timescales. Quantum metrologists seek to improve upon traditional metrological methods by looking for ways of making high-resolution measurements of physical parameters using quantum theory and trying to link measurements to nature’s fixed fundamental constants.
Uncomplicated relationships?
In the 1960s Brian Josephson discovered that when a junction between two superconductors is irradiated with microwaves, the voltage that appears across the junction is proportional to Plank’s constant (h) and inversely proportional to the electron charge (e) – two fundamental constants of nature. Since the voltage is not affected by the dimensions of the junction or the materials it is made of, this voltage standard can be reproduced anywhere at any time and will always be the same.
Similarly, a quantum standard for resistance, known as the quantum Hall effect, was defined by Klaus von Klitzing 15 years later. He found that putting a superconducting material at a temperature of almost absolute zero in a magnetic field 100,000 times stronger than the Earth’s renders the superconductor’s resistance independent of the properties of the material, and again only dependent on e and h.
But, explains Bernd Kaestner of the Physikalisch-Technische Bundesanstalt, Germany, who helped build the new chip, “There is an uncertainty to e and h that is still quite large, so one can never be sure whether the quantum Hall effect and the Josephson effect are absolutely determined by these quantum relations or whether there are small corrections to them.”
Trouble with triangles
For the last 20 years, scientists have been attempting to link these two quantum-electrical standards in a triangle, defining a quantum-current standard in terms of e and h – the single-electron transport effect – as the third arm. If realized, this quantum metrological triangle would be able to test the consistency of the three electrical standards and work out whether any of the relations are in need of some fine-tuning.
“Any renormalizing factor would be incredibly small,” explains J T Janssen of the UK’s National Physical Laboratory, who was not involved in the research, “but it would also be incredibly important, because it would undermine the existing theory.”
Single chip approach
Kaestner and colleagues designed a chip that would generate discrete quantized voltages, by placing a semiconducting single-electron pump and a quantum Hall device in series. The voltage generated was dependent only on the current, which in turn was dependent only on the frequency of the single-electron pump.
“So you have now two sides of the triangle, if you like, combined in one device,” explains Kaestner. This offers an independent check on the Josephson voltage, because the Josephson effect relies on superconductor physics, and the new device on semiconductor physics. “Now one can [try to] produce exactly the same voltage with two fundamentally different physics…This is one elegant way of closing the triangle.”
Simplifying and scaling
“It is certainly a nice experiment to make these two quantum standards in one device,” comments Janssen. But the voltages it generates are only of the order of microvolts. Because quantum Hall devices produce noise in the region of nanovolts, he cautions “You would have to measure for a very long time to get any sort of resolution.”
The researchers, on the other hand, suggest that their device is easily scalable, which would allow the signal-to-noise ratio to be brought down significantly. Kaestner argues that an integrated circuit is also the most logical approach, since each micron-sized chip must be kept at a given temperature – about 1 K in this case – by cryostats that occupy several cubic metres of lab space.
But Janssen questions the practicality of connecting 10 current sources and 100 quantum Hall devices in series on one tiny chip, as the researchers propose. His team favour a current amplifier, which ramps the current from a separate single-electron device by a factor of about 10,000 without introducing much error.
“It is a nice experiment they have done, it is a nice device, but it would be very difficult for this device to compete with the traditional technology that we are using at the moment…there would have to be a significant breakthrough in device replication to make this competitive,” he says.
Kaestner hopes that this initial proof-of-principle work will prompt others to think of new and innovative combinations of integrated quantum devices. “Single-electron sources are very new, and I think the semiconductor industry is not aware of the possibilities,” he explains, adding “this is really a new circuit component.”
The research is published in Physical Review Letters.