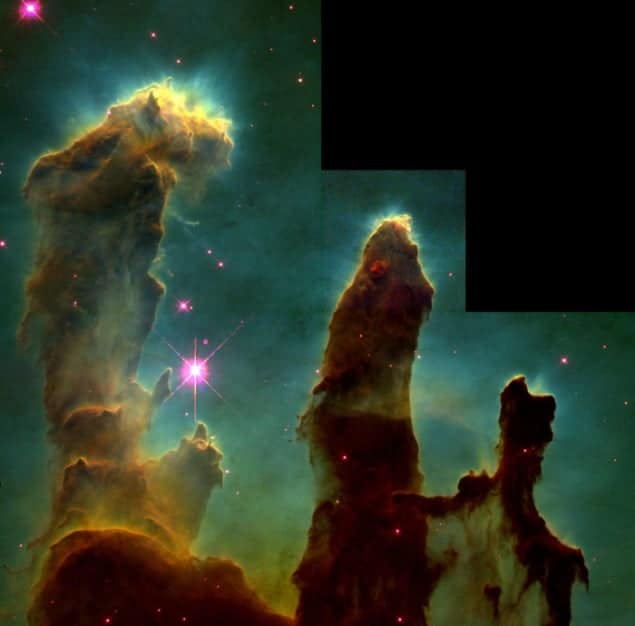
Black-body radiation can give rise to a net attractive force between tiny objects. That is the claim made by physicists at the University of Innsbruck in Austria, who have calculated the strength of this new force between a speck of dust and a hydrogen atom. The team believes that in some situations the force could be more significant than gravity – which means that its presence could have important effects on the behaviour of clouds of gas and dust in space.
Pulling things with electromagnetic radiation – the so-called tractor beam – has long been a mainstay of science fiction. While physicists have enjoyed some luck creating specialized systems to achieve it, any system must overcome a fundamental challenge: a particle absorbing a photon is pushed, not pulled.
Incident radiation can affect an atom in two ways. If the photon has precisely the right energy, then it can promote an electron into an excited atomic state. As the atom absorbs the photon, it also absorbs its momentum. This pushes the atom away from the light source, causing radiation pressure. More subtly, the electric field of the light wave causes its energy levels to shift slightly – a process called the Stark effect. While some excited states are raised in energy, the ground state is generally lowered.
Attracted to radiation
Unless the atom has recently been excited by a photon, then the electrons will be in the ground state; so reducing its energy reduces the total energy of the atom. More intense radiation creates a stronger electromagnetic field and a larger Stark shift, so the natural tendency of atoms to minimize their energy creates an attractive force towards the source of the radiation. This force is used by optical tweezers to trap atoms at a laser focus.
Theoretical physicist Helmut Ritsch of the University of Innsbruck explains that the current work arose from a speculative discussion with his wife, biomedical physicist Monika-Ritsch Marte, who studies optical tweezers at the Medical University of Innsbruck. They pondered whether or not an attractive optical potential could be created using broadband light. “Most people would first say no,” he explains. The pair teamed up with PhD student Matthias Sonnleitner to study the case of black-body radiation – the most broadband light imaginable.
Stark contributions
The black-body radiation emitted by an object contains a continuous spectrum of frequencies, so the photon energies required to excite atoms will be present, and these photons create a repulsive force. However, the energies of most common atomic transitions, at least in the lighter elements that make up most of the universe, correspond to photon frequencies in the visible or ultraviolet region of the electromagnetic spectrum. Radiating black bodies with temperatures below about 6000 K – the temperature of the surface of the Sun – emit the vast majority of their radiation as infrared photons. Because these photons have an energy below that needed to excite the electronic transitions they are not absorbed and do not cause radiation pressure. They do, however, contribute to the attractive force created by the Stark effect. In most physically realistic cases, therefore, this attractive “black-body optical force” is greater than the radiation pressure.
The force decays rapidly with distance and therefore the researchers believe that it will be challenging to measure in the laboratory. Under specific astrophysical conditions, however, it may play a key role. It is likely to be most significant for objects that, while below the temperatures of thousands of Kelvin necessary for radiation pressure to become significant, are hot enough to radiate appreciably. Moreover, in particles that are very light, it could be more significant than gravity. For example, modelling of an interplanetary dust cloud of micrometre-sized particles at 100 K suggests that the black-body potential at its surface is more than 100 million times the potential caused by gravity.
Astrophysical feedback
Helmut Ritsch now hopes to explore the detailed implications of the model in various scenarios. “We have certainly got a lot of feedback from the astrophysics community,” he says. “They have suggested a few scenarios that we should look at.” He says, for example, that immediately after the first hydrogen formed in the early universe, there would still have been plenty of radiation free, so radiation-mediated binding between hydrogen could perhaps have altered the evolution of density fluctuations.
Miles Padgett, an optical physicist at the University of Glasgow, is enthusiastic. “I think it is lovely,” he says. “It is a new mechanism completely different from all the others that have been previously discussed in the optical-trapping community.” He believes that, under high vacuum and on a small scale, it may be possible to detect the force directly in the laboratory.
Theoretical atomic physicist Andrei Derevianko of the University of Nevada says that, in principle, the attractive effect of electromagnetic radiation had previously been known in theory and used in practice in specific cases but that spelling out the full implications may have a significant impact. “This whole subject of atom–light interactions is quite exotic,” he says, “It is not like standard science. You really have to come in and have somebody say that this could have astrophysical implications for the other community to become aware of it. Work like this builds bridges.”
The research is described in Physical Review Letters.