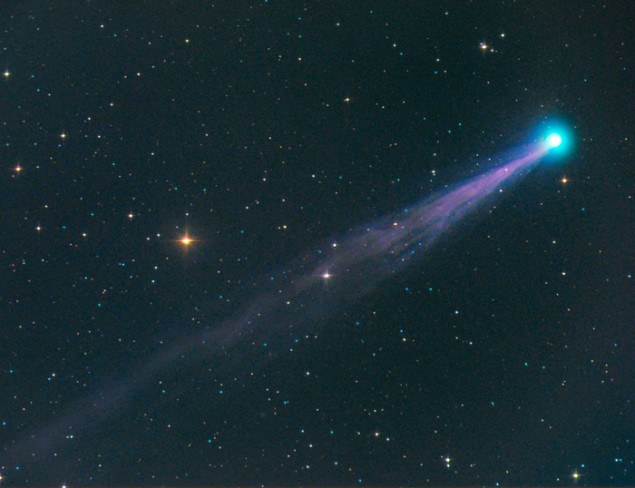
Certain carbon-based molecules could be used to trace extreme pressure and temperature environments in the universe that are caused by supernovae or colliding planets, thanks to new research carried out by scientists in the UK. According to the team, studying aromatic hydrocarbons, which are often found in meteorites, could allow us to investigate imprints left on the hydrocarbons caused by violent events in our universe’s past.
Carbon is the fourth most abundant element in the universe after hydrogen, helium and oxygen. The relative ubiquity of carbon means that a variety of carbon-bearing molecules have been observed in comets, asteroids and planets, including the sample of comet material that was returned to Earth in 2006 from NASA’s Stardust mission. “The cosmos is replete with carbonaceous material,” says Wren Montgomery, a postdoctoral researcher at Imperial College London who led the new study. In the past, however, researchers have only been able to study how such molecules are affected by heat. “There are lots of organic markers of temperature in the cosmos but none for pressure,” Montgomery explains.
Now, the researchers have conducted laboratory experiments on how the spectral signatures of aromatic hydrocarbons – molecules that contain benzene rings and characteristically have a sweet odour – change in response to pressure. The researchers used dimethylnaphthalenes – hydrocarbons that are also relatively simple to analyse spectroscopically and that have been shown to exist in carbonaceous dust in the interstellar medium.
Squeezed by diamonds
“Heat is not the only modifier of organic matter in the cosmos. Pressure is also a major agent of change and the variation in pressure in space is extreme,” says Montgomery. Supernovae can reach maximum pressures of 103–106 GPa, hundreds of billions of times higher than terrestrial atmospheric pressure. Such intense pressure environments alter the molecular structure of dimethylnaphthalenes. Montgomery and her team subjected samples of dimethylnaphthalene isomers to pressures ranging from 0.5–21 GPa using a diamond-anvil cell, a device that uses two diamond faces to exert high pressures over a small area (typically a few hundred microns in diameter). The researchers performed mid-infrared spectroscopy using synchrotron light and recorded the wavelengths of characteristic peaks that appeared in the spectra over 9.5–14.0 μm. The experiments were repeated at both the Swiss Light Source in Villigen, Switzerland, and at SOLEIL, the French national synchrotron facility near Paris.
Spectral shifts
The researchers found that the dominant spectroscopic features of the isomers characteristically shifted to bluer wavelengths with increasing pressure. The blueshifts were approximately 0.5–0.75 μm, which the team attributed to increasing energy from the compression of nuclei and electrons. However, one of the dimethylnaphthalene isomers – 1,5-dimethylnaphthalene – showed little change in its spectrum up to pressures of 18.1 GPa. This same isomer shows distinct spectral changes as a function of temperature, which opens up possibilities for using 1,5-dimethylnaphthalene to discriminate between the effects of pressure and temperature. The team says that the observed responses, showing the pressure stability but thermal instability of 1,5-dimethylnaphthalene, could be used as an indicator of organic modification. In the coming months, the team plans on subjecting other types of aromatic hydrocarbons to a range of pressures that are experienced in space, and so will build up a comprehensive catalogue of all aromatic hydrocarbons to understand more about high-pressure zones.
The team proposes that the information contained in the molecular structure of dimethylnaphthalenes can be collected using the existing technology on board roving laboratories, such as the one on the Mars Science Laboratory Mission. Tracing the barometric history of hydrocarbon molecules can shed light on how carbonaceous material was processed to ultimately form planets.
The research is published in The Astrophysical Journal.