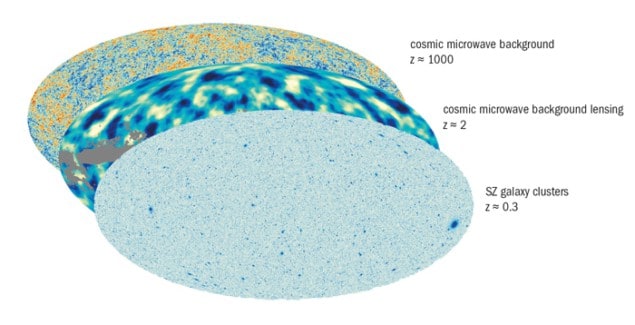
Scientists know that when they measure the total amount of matter in the universe using two competing methods, one will give a higher value for the total density of matter than the other. To resolve this measurement discrepancy, two separate research groups have now proposed that the missing mass might be in the form of neutrinos. Accurately measuring the total amount of matter in the universe is a crucial cosmological parameter for interpreting a vast number of astrophysical phenomena.
Neutrinos are difficult to study because they interact only by the weak nuclear force, which acts only over very short distances, and by gravity, which is extremely weak. In the Standard Model of particle physics, neutrinos come in three flavours – electron, muon and tau. They were once thought to be massless, but the discovery of neutrino oscillations – whereby neutrinos change flavour, requiring that their masses be different – implies at least two of them have mass, with the rate of oscillation depending on the mass difference of each flavour. The rate has been measured in particle-physics experiments such as SuperKamiokande in Japan, which has allowed particle physicists to place a lower bound on the sum of the neutrino masses of 0.06 eV, but the absolute values remain unknown.
Cosmic discrepancies
Measurements of the total amount of matter in the universe that are made based on cosmic microwave background (CMB) – the thermal remnant of the Big Bang – generally give a higher value for the total density of matter, as compared with the measurements that look at the number of galaxy clusters and gravitational lensing. In the first of the new papers, Richard Battye of the University of Manchester and Adam Moss of the University of Nottingham, both in the UK, suggest that massive neutrinos could account for the fact that the total mass inferred from the size of ripples in the CMB – measured by satellites such as Planck and the Wilkinson Microwave Anisotropy Telescope (WMAP) – is higher than the total mass measured by the other methods. Mark Wyman and colleagues at the University of Chicago in the US make the same suggestion based on different data sets in the second paper.
The CMB came into being about 380,000 years after the Big Bang, when neutral atoms first formed and the decoupling of matter and radiation allowed photons to travel freely across the universe. Neutrinos at this temperature (about 3000 K) would have been highly relativistic. Now, however, they have cooled down to the point where their mass is effectively their rest mass. They could therefore account for the apparently lower mass of the universe today than in the CMB.
The number of neutrinos produced when neutral atoms first formed can be calculated relatively easily, and if the sum of the neutrino rest masses is 0.06 eV, neutrinos would make a negligible contribution to the mass in the universe. The authors of the two papers therefore calculated what combined mass would actually reconcile the CMB observations with observations of the universe made today. Both groups considered two possibilities – the first, and simplest, involved adding to all the masses of the known neutrinos. In this case, Battye and Moss calculated a combined mass of approximately 0.32 eV and the Chicago scientists arrived at a figure of about 0.39 eV (well within each other’s errors).
Sterile particles
Both groups also looked at models containing “sterile neutrinos” – a proposed and much-debated fourth type of neutrino that contributes mass but does not interact with the weak force. Such sterile neutrinos would therefore only interact with the other three “active neutrinos”, making them that much more difficult to detect.
In the new work, the researchers kept the active neutrino mass pegged at 0.06 eV and attributed the extra mass to sterile neutrinos. Depending on the exact model they used, Battye and Moss found a sterile-neutrino mass between 0.3 eV and 0.5 eV, while Wyman’s group found the mass would be around 0.4 eV. This model fitted slightly better with measurements of the matter in the universe made using other techniques than scenarios with heavy active neutrinos. “In our paper we were fairly even handed between the two situations,” says Wyman, now at New York University, “but we chose to present our figures for the sterile-neutrino case because, in our collaboration, we all view that as the more likely case from a particle-physics standpoint.”
Observational cosmologist Ofer Lahav of University College London, who in 2010 placed an approximate upper bound on the neutrino mass of 0.28 eV, is intrigued but sceptical. “It’s extremely interesting to get the neutrino mass from cosmological data sets,” he says. “It’s also very challenging – I can easily see different analyses getting slightly different answers, and equally I think the tension in the data might have other explanations such as local variation of the Hubble constant or other systematic errors.”
Both papers are published in Physical Review Letters (Phys. Rev. Lett. 112 051303; Phys. Rev. Lett. 112 051302).