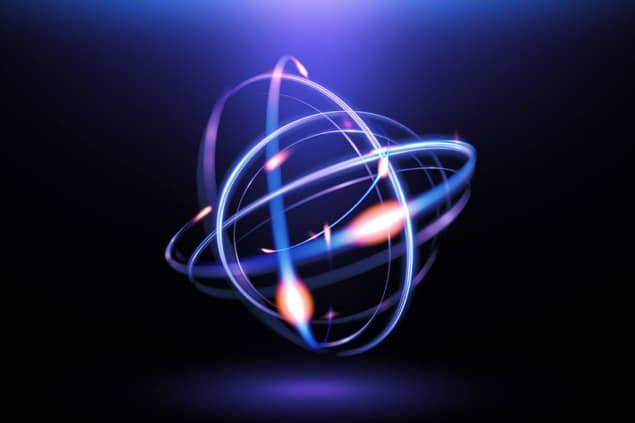
An excited atom decaying in a vacuum experiences a force very similar to friction, according to calculations done by physicists in the UK. At first sight, the result appears to violate Einstein’s equivalence principle. However, the researchers calculate that, in fact, relativity rides to its own rescue, and the mass lost from the atom as it decays to the ground state allows it to lose momentum without slowing down.
Einstein’s special theory of relativity famously says there is no such thing as absolute motion: the laws of physics are the same in all inertial frames of reference. Theoretical physicists Matthias Sonnleitner, Nils Trautmann and Stephen Barnett at the University of Glasgow noticed an apparent contradiction, however, when considering a textbook quantum-mechanics problem.
An excited atom in a vacuum decays to a lower energy state, emitting a photon in a random direction. For simplicity, the problem is normally solved in the rest frame of the atom. In this frame, the magnitude of the photon’s momentum is independent of its direction so, as a photon is equally likely to be emitted in any direction, the expectation values of the photon’s momentum and the atom’s consequent recoil momentum remain constant at zero.
Net force
However, the trio also considered the problem in a frame in which the atom is moving: because of the Doppler effect, a photon emitted in the same direction of travel as the atom would be blue-shifted, having its frequency, and therefore its momentum, increased; whereas a photon emitted in the opposite direction would be red-shifted and have its momentum decreased. The atom would therefore experience a net force proportional to its momentum but in the opposite direction – effectively, it would experience friction from the vacuum.
This appears to violate the principle of relativity because, if the atom’s velocity changed, an observer could measure this change in velocity and use it to determine the absolute motion of the observer’s own frame of reference. Sonnleitner says the trio spent “weeks questioning their sanity”. They later discovered an earlier paper, published on the arXiv pre-print server in 2012, in which Wei Guo of Queens University of Charlotte in North Carolina identified the problem but could not solve it.
The Glasgow researchers eventually realized that, although they had not explicitly included relativity in their calculations, it had nevertheless sneaked in the back door: when an atom emits a photon and decays to a lower energy state, the classic equation E = mc2 shows that its mass must also decrease. Although the decrease is tiny, it is precisely sufficient to compensate for the decrease in momentum, allowing its velocity to stay constant. This only works when a small, often-neglected correction proposed in 1888 by the physicist Wilhelm Röntgen (who won the 1901 Nobel Prize for the discovery of X-rays) is included to accommodate the interaction between the moving atom’s electric dipole and a magnetic field. In this latest research, the magnetic field is associated with quantum vacuum fluctuations. “[Guo’s 2012 paper] dropped the Röntgen term at some point,” explains Sonnleitner. “The Röntgen term is necessary to get the correct change in momentum. Only then do you see that it’s just due to a change in mass and not due to a change in velocity.”
Immeasurably small
Although the researchers considered the simplest possible situation, in which one atom in a vacuum decays by photon emission, the phenomenon is, in principle, applicable whenever an atom absorbs or emits a photon. “If this effect were larger,” says Sonnleitner, “You would see its contribution whenever you tried to cool an atom, for instance.” In practice, however, other influences are much larger in these cases, so the effect is not significant. He adds, “Experiments are getting so, so much better right now that it’s really hard to say that something cannot be measured at all, but at least as far as I’ve seen this one is not feasible yet.”
“This is an interesting conceptual point,” says theoretical physicist Peter Milonni of the University of Rochester in the US. “Maybe this work will lead the way to experiments to probe this conceptual difference between the change in momentum associated with translational motion and the change in momentum associated with the internal energy dynamics – these kinds of things are well known in nuclear physics. Whether it will lead to practical consequences in the theory of laser cooling and trapping, and new ways to trap atoms and so on: I don’t think so, but I could be wrong.”
The research is described in Physical Review Letters.