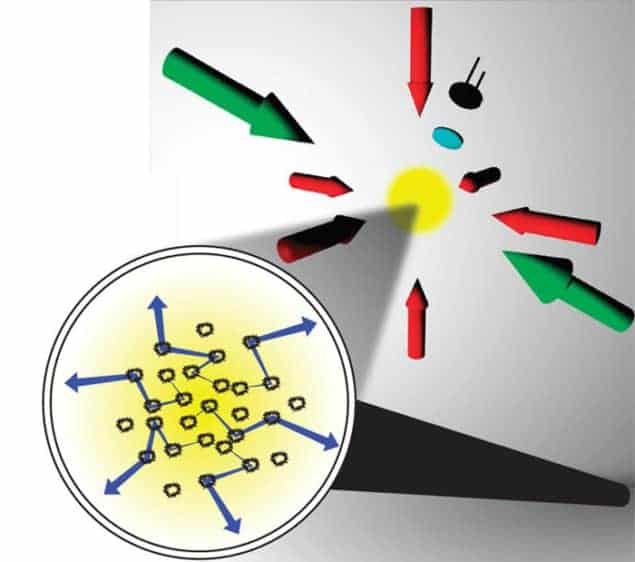
Physicists in France are the first to create a random laser in a cloud of cold atoms under laboratory conditions. The effect was first seen decades ago in stellar clouds and the team believes that its set-up could provide basic insights into the conditions necessary for random lasing. This could improve our understanding of astrophysics and even lead to practical applications of the phenomenon here on Earth.
A conventional laser usually comprises a gain medium (solid, liquid or gas) that is sandwiched between two mirrors. Light bounces back and forth many times in this optical cavity, stimulating the emission of more light and creating a coherent field of light. In a random laser there are no mirrors and the light simply bounces around between particles located at random positions in the gain medium. This light can stimulate the emission of light from the medium just as in a conventional laser. However, because of the random paths taken by the light, a laser beam is not produced. Instead, coherent light is emitted in all directions.
Random lasing was first proposed in the 1960s to explain why certain specific emission lines in some clouds of stellar gas are more intense than theoretically predicted. On Earth, random lasers have been made using liquid suspensions and solid powders. In these lasers, the light-scattering particles are classical objects such as grains of zinc oxide – whereas astronomers believe that atoms do the scattering in stellar-gas lasers.
Forbidden transition
Robin Kaiser and colleagues at the CNRS Non-linear Institute of Nice made their laser with a cloud of cooled rubidium-85 atoms confined to a magneto-optical trap. They used a pump laser to create a population inversion between two hyperfine levels of the same electronic orbital and a second, tunable laser to stimulate emission back to the lower level. The key to creating a random laser is to ensure that some of this emitted light will be scattered by the atoms, rather than being reabsorbed by them. This was done by adjusting the wavelength of the tunable laser such that the emitted light corresponded to that of a forbidden transition in the atom. The researchers found that, when the laser light had exactly the same frequency as the forbidden transition, there was a bump in the intensity of the laser output – a sign that it was being boosted by random lasing.
This is the first demonstration of a random laser in the laboratory in which photons are scattered by atoms, as happens in stellar gases. Whereas the effect of radiation pressure on a grain of zinc oxide is insignificant, the scattering of a photon will cause measurable recoil in an atom. Whether or not this and other effects of random lasing have macroscopic effects in astrophysics remains an open question, however. “It’s possible in principle that, if you put more stimulated emission into the radiation, the sign of the radiation pressure could be inverted,” Kaiser explains, “so we could have attractive components of the radiation pressure.” He points to the team’s observation of an oscillating cloud of cold atoms bears similarities to Cepheid variables, an oscillating equilibrium between gravity and radiation pressure. So signs of negative radiation pressure should be accessible to experimental observations.
Kaiser hopes that such ideas might be testable in the laboratory with a gaseous random laser. He points out, however, that significant differences between the team’s laboratory device and a stellar-gas laser remain. The atoms in their magneto-optical trap are cooled to about 50 µK, whereas the atoms in a stellar gas are hot. The team pumped the random laser with another laser, while a stellar gas is pumped by broad spectrum light from a star. Kaiser hopes to optimize the team’s system to resemble more closely the conditions in a stellar environment. “I want to interact more with people from astrophysics to find out which schemes are more or less realistic,” he says.
Quantum effects
Diederik Wiersma, an expert on disordered photonics at the University of Florence, is impressed by the work. He believes that, in addition to radiation pressure, it may be possible to study other phenomena, such as the effect of photon scattering from several atoms in an entangled state and quantum interference between atoms and photons. “You might have access to knowledge about which path the photon took,” he explains, “and if you have that, quantum mechanics tells you it will not behave as a wave anymore.”
Further into the future, Wiersma suggests that the system may aid understanding of quantum computing. “There have been proposals about a possible quantum Internet,” he says, “where you could use optical connections to connect matter at different locations in a single quantum state.”
The research is published in Nature Physics.