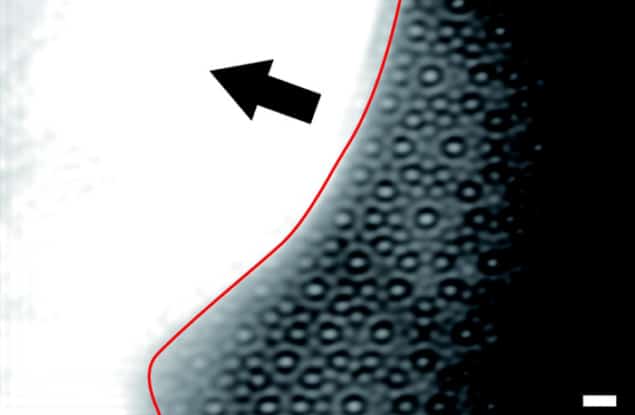
The first “movies” of quasicrystal growth reveal that an unexpected error-correction process is involved in creating the curious structures. Taken by physicists at the University of Tokyo, the high-resolution images suggest that short-range interactions dominate the quasicrystal formation process – rather than long-range interactions, as had been previously thought.
Conventional crystals are characterized by repeating unit cells of atoms, which combine to create a simple crystalline structure with translational symmetry. This symmetry means that if an observer were to move from one unit cell to another, the surrounding crystal would look exactly the same. Such crystals grow via short-range, local interactions between unit cells, because growth consists of simply repeating a single pattern.
Quasicrystals, on the other hand, can be modelled as two unit cells – called Penrose tiles – that repeat in a more complicated way to create a structure that has rotational symmetry but lacks translational symmetry. The growth of quasicrystals appears to require atoms to have long-range, nonlocal interactions with each other to create these complex patterns – something that does not have a physical explanation. The problem is that each atomic cluster in a quasicrystal should only have information about its immediate neighbours, and should therefore lack the long-range information necessary for quasicrystalline patterns to form. Indeed, many prominent scientists, including the Nobel laureate Linus Pauling, dismissed the idea of quasicrystals when it first emerged.
Open question
While there is now little doubt that quasicrystals exist, how they grow has remained an open question since they were first discovered in 1984 by Daniel Shechtman, who bagged the 2011 Nobel Prize for Chemistry for his pioneering work on the subject.
Our observations reveal a peculiar process of quasicrystal growth that is very different from the ideal growth models
Keiichi Edagawa, University of Tokyo
Today, quasicrystals are used in everyday objects such as frying pans, surgical scalpels and razor blades, but their growth had remained poorly understood. Now, Keiichi Edagawa of the University of Tokyo and his team have used high-resolution transmission electron microscopy to directly observe the growth of a quasicrystal that is an alloy of aluminium, nickel and cobalt. “Our observations reveal a peculiar process of quasicrystal growth that is very different from the ideal growth models proposed so far,” Edagawa told physicsworld.com.
Growing grain
Edagawa and his team made an Al70.8Ni19.7Co9.5 quasicrystal with 10-fold rotational symmetry, meaning that rotating the pattern by integer multiples of 36° gives an identical pattern. The researchers heated the sample to a temperature of 1183 K, which caused atoms from one grain in the quasicrystal to detach and join another grain. The researchers used a high-resolution transmission electron microscope to record a movie of grain growth at 30 frames per second. “Our resolution is sufficient to resolve atomic clusters,” Edagawa says.
The team observed that errors were frequently introduced into the structure as the atomic clusters arranged themselves. However, these errors were consistently corrected over the course of a few seconds to restore the quasicrystalline order. Observing these errors was a surprise: theoretical models of quasicrystal growth had predicted that grains grow perfectly and always preserve the quasicrystalline order.
Six instances of “error-and-repair” events were observed over 15 s on one grain. The presence of these errors suggest to Edagawa and his team that the growth of quasicrystals is indeed governed by local atomic interactions; nonlocal interactions would ensure constant quasicrystalline order and obviate the need for error-and-repair events. “Local interactions appear to suffice to construct ideal quasicrystalline order,” the researchers reported, an assertion that is supported by recent Monte Carlo and molecular-dynamics simulations. The team believes that the assembly errors increase the free energy of the quasicrystal and that the subsequent rearrangements back to the quasicrystalline order decreased the free energy again.
The observations are described in Physical Review Letters.