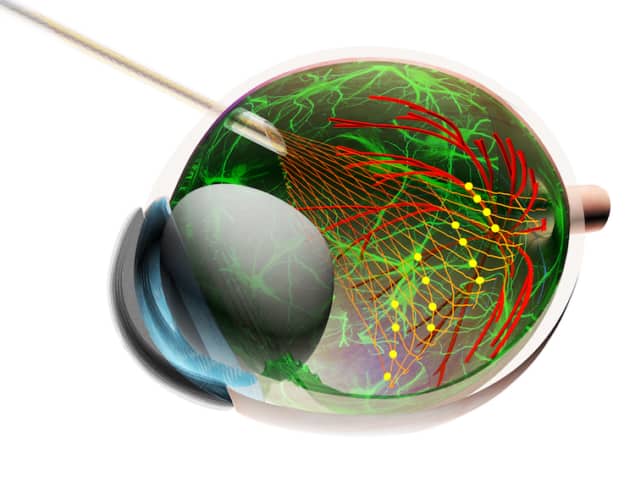
Mesh electronics, a macroporous network of components with mechanical properties similar to that of biological tissue, is a relatively new technology that can be used to probe activity in the brain. Now, researchers at Harvard University in the US have developed an injectable mesh that can record the neural activity of mouse eyes in vivo. The device, which does not interfere with eye movement or light-processing, could help neuroscientists study the fundamental properties of primary vision input retinal ganglion cells (RGCs) and how these cells connect with other vision-related brain regions for the first time. The work could also help in the development of retinal prosthetics for restoring vision through non-surgical procedures.
“Mesh electronics is a submicron-thick, large-area macroporous network,” explains team leader Charles Lieber. “We fabricate the meshes as flat 2D sheets using standard semiconductor photolithography-based techniques and suspend them (like a colloid) in aqueous solution. Our specific design, which we first reported on back in 2015, enables mesh electronics to be rolled up into a tubular structure and drawn into a syringe needle.”
On the scale of a single neuron
“We can deliver these structures into specific brain regions with a spatial precision of 20 microns (which is on the scale of a single neuron) using the controlled injection approach we developed. This allows us to control the rate at which we withdraw the needle during injection and means that the mesh structure remains fully extended in the dense tissue of the brain during injection and does not crumple.”
In their new work Lieber and colleagues “non-coaxially” injected the mesh electronics onto the highly curved retinal cup of the eye. As the structure unrolls it forms a stable recording interface to RGCs, which process visual information received by photoreceptors (rods and cones). The researchers then did a series of experiments.
Monitoring the activity of the RGCs
“First, we monitored the activity of the RGCs using the device and were able to measure the response of different subtypes of these cells in a chronically stable manner,” explains team member Guosong Hong. “We found that some RGCs respond to light intensity while others respond to other visual cues, such as gratings comprising alternating black-and-white stripes and moving in specific directions. We were able to monitor individual RGCs repeatedly over a two-week period.
“Next we monitored the RGCs at four-hour intervals over several day-night cycles and found that some of the cells fire with a higher activity during the day-time circadian phase than during the night time phase, while some others do the opposite. This reveals, for the first time, the idiosyncrasy of circadian behaviours of different RGCs.”
The retina, which processes visual information and sends it to the brain, is an excellent model for studying neural circuitry. Until now, however, most retina studies meant killing laboratory animals and then removing their retinas to analyze. This meant that the information obtained was limited.
First high-resolution measurement of the retina in awake animals
“Existing techniques to measure in vivo neural activity in retinas typically make use of metal microwire electrodes to record from a few RGCs in anaesthetized animals with relatively large eyes over a short period of time,” says team member Tian-Ming Fu. “Or they rely on electroretinography (ERG) to measure the collective activity of a population of neurons in the retina with limited spatiotemporal resolution. Our method is the first high-resolution chronic measurement of the retina in awake animals.”
The researchers, reporting their work in Science DOI: 10.1126/science.aas9160, now have several new projects under way. “For one, we would like to map the entire visual pathway, from the retina through to the lateral geniculate nucleus (LGN), which is a relay centre in the thalamus, to the visual cortex and higher-level brain regions at the single cell level,” Lieber tells Physics World. “We would also like to apply the non-coaxial mesh electronics injection technique, demonstrated for the first time in this work, to other parts of the nervous system, such as the spinal cord and the neuromuscular junction.”