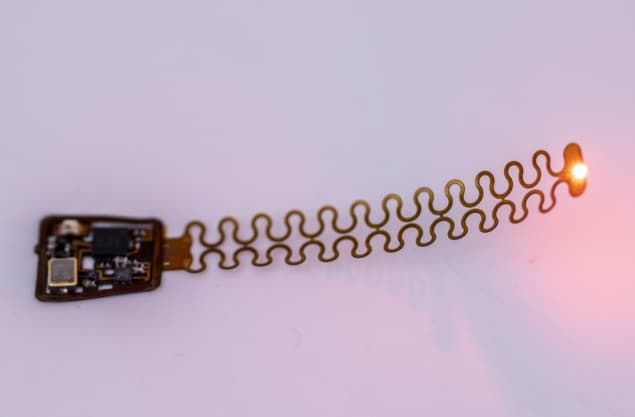
Researchers in the US have designed a completely implantable, wireless, battery-free oxygen sensor to monitor tissue oxygen levels when implanted subdermally or even in deep brain regions. With these features, the newly fabricated oximeter supports in vivo tissue oxygen monitoring in awake and free-moving animals such as mice (Science Advances 10.1126/sciadv.aaw0873).
Oxygen levels in different regions of tissue represent the balance between oxygen demand and supply. Imbalance and abnormalities in tissue oxygen levels are of relevance to various physiological or pathological processes, such as neural activity, tissue perfusion, the tumour microenvironment and wound healing. Therefore, determining tissue oxygenation is of significant importance.
To ascertain regional tissue oxygen levels, existing methods either measure oxygen partial pressure or assess changes in the concentration of oxygenated haemoglobin. However, most approaches developed to date interfere with the natural behaviours of the test subjects (for example, by requiring physical tethers or anaesthetics). Unfortunately, these limitations can alter oxygenation levels and lead to inaccurate oxygen measurements. In addition, depth of operation in several tissues has remained a challenge for many existing oximeters.
Design and working principle
To overcome these restrictions and challenges, the researchers — led by John Rogers at Northwestern University — designed a thin, fully implantable wireless oximeter. Their design contains an injectable filamentary measurement probe connected to an electronic module. The filamentary probe performs the optoelectronic measurements, while the electronic module supports wireless data communication.
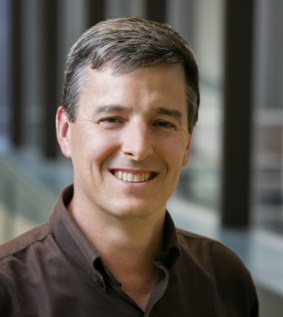
The sensing probe exploits differences in the optical properties of oxygenated haemoglobin (HbO2) and deoxygenated haemoglobin (Hb) to infer local changes in their concentrations. This quantification is then used to estimate regional tissue oxygen saturation (rStO2) levels.
The estimation of rStO2 levels depends on the absorption spectra of both oxygenated and deoxygenated haemoglobin in the visible and near-infrared spectral range. At high oxygen concentrations, the ratio of HbO2/Hb increases and at low oxygen concentrations (hypoxia), it tends to decrease.
Sensitivity to oxygenation of haemoglobin is manifested in the differences between the molar extinction coefficients of HbO2 and Hb. The measurable optical properties, such as light attenuation by haemoglobin, then define the rStO2 as a function of HbO2 and Hb concentrations.
Wireless data communication occurs via magnetic resonant coupling-induced power harvesting and infrared-enabled data transmission. To improve stable operation in chronic implants, and also shield the devices from biofluids, the researchers used bioinert coatings in which a conformal coating of parylene surrounds the device.
Device characterization
To assess the device’s functionality, the researchers tested their system in vivo, in deep brain regions of both anaesthetized and free-moving mice, as well as in artificial blood solutions. They observed that the assembled optoelectronic platforms demonstrated continuous, sensitive and localized rStO2 sensing at regions-of-interest.
The fabrication concepts and electronic designs could also enable implantable platforms with other functionalities, such as heart rate tracking. “Other extended options include the integration of the oximeter probes with other functional modules for optogenetic modulation or microfluidic drug delivery,” the authors speculate. “These multimodal systems with colocalization of stimuli and oxygenation detection could support unique capabilities in coupling the metabolism of specific tissue regions with external physiological or pathological challenges.”