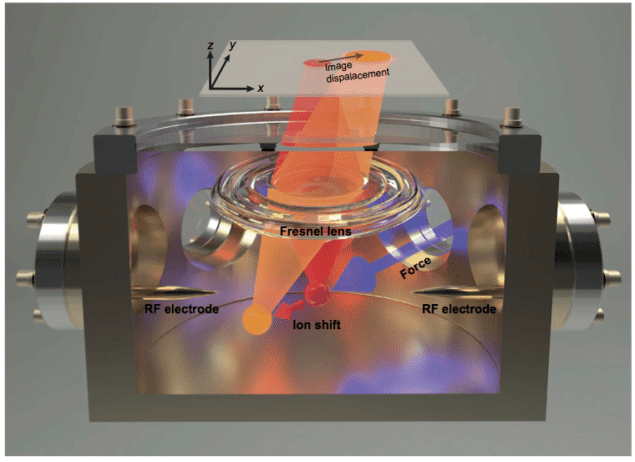
A 3D force sensor that can image single trapped ions with a resolution on the sub-attonewton scale could be used in a number of practical areas, including as a probe to detect the electric fields surrounding biomolecules and nanoparticles, and to investigate surface properties. The new sensor is very similar to a recently demonstrated atomic microscope force sensor but it has a substantially higher density and potential for further improvement.
Measuring force with high sensitivity is important for investigating the fundamental physics of magnetic, atomic, quantum and surface phenomena. It is also important for precisely measuring physical quantities, such as the effect of gravity on time in the theory of general relativity.
In recent years, researchers have developed high-resolution imaging techniques based on laser-cooled trapped ions that have opened up the possibility of making ion-based sensors that could resolve an external force in three directions using a single atomic ion. This is thanks to Hooke’s law Fi = kiΔxi, which allows to convert displacement measurements Δxi into a force measurement Fi through the associated spring constants ki.
Paul trap
The new ion trapping apparatus made by Erik Streed and colleagues of the University of Brisbane in Australia consists of a single 174Yb+ ion confined in a so-called Paul trap formed in a 3D quadrupole created by two radio-frequency-excited tungsten needles. The researchers cool the ion down to 0.5 mK using laser radiation with a wavelength of 369.5 nm. They resulting fluorescence (which also has a wavelength of 369.5 nm) is collected using a binary phase Fresnel lens and imaged onto a cooled electron-multiplying charge-coupled device camera (EMCCD) with a total magnification of 395.9 ± 0.6 nm.
Streed and colleagues are able to measure full width at half maximum (FWHM) diameters in the image of their trapped ion of 378 and 393 ± 1 nm in the x and y directions respectively. The researchers attribute most of the error in their measurement to mechanical drift because they did not specifically engineer their system to be stable for long periods on the nanometre scale. This situation can be readily improved with a suitably designed system, they say.
With a 20 second exposure time (and accounting for systematic drifts), they can determine the ion’s centroid position with a precision as high as 2.8 and 10 nm in the x and y directions.
Atom-sized version of a ball attached to a spring
“Our force sensor is essentially the atom-sized version of a ball attached to a spring,” explains Streed. “Since our atom is missing an electron, it is electrically charged and therefore very sensitive to electric fields. When we apply an electrical force, it thus pushes the atom so that it changes its position. We know how tightly the atom is being held in the trap and can therefore very accurately measure (on the scale of nanometres) how much it has moved. We can subsequently calculate the magnitude of the force (spring constant) that pushed it.”
The Brisbane team measured spring constants of 7.29 ± 0.02, 7.83 ± 0.02, and 29.22 ± 0.04 zN/nm at measured trap frequencies of 800 ± 1, 829 ± 1, and 1601 ± 1 kHz respectively using its technique.
3D force sensing
When in focus, the ion image is insensitive to motion along the third, z, direction so the researchers had to use a trick to be able to measure force in 3D. “Generally, when you take a picture of an object, you can only see it in 2D. Our trick consists of moving the atom a little bit out of focus, and then measuring if it is becoming more out of focus or more in focus to calculate how much it has moved in the third dimension.”
Since we are measuring the force at what is nearly a single point, we do not have to be concerned about the systematic problems that occur when there are multiple atoms involved, Streed tells nanotechweb.org. “Previous sensors like this one worked using the Doppler effect, so ions needed to be moving for us to be able to detect the force. What is more, the force needed to be at a frequency close to the one that the ions naturally wanted to move at. This made averaging more difficult.
Longer camera exposure produces a better image
“In our case, if we want a more sensitive measurement, all we need to do is take a longer camera exposure to obtain a better image of the ion, and so determine its average position with a higher accuracy.”
So, what about possible applications? “I am also a biophysicist so am interested in using this sensor to probe the electric field surrounding individual biomolecules as they are unravelled,” says Streed. “My colleagues and I would also like to probe surfaces with it, mostly of other types of ion traps, because it is these surfaces that limit their performance for applications such as quantum computing.”
The new 3D sub-attonewton force sensor is detailed in Science Advances 10.1126/sciadv.aao4453.