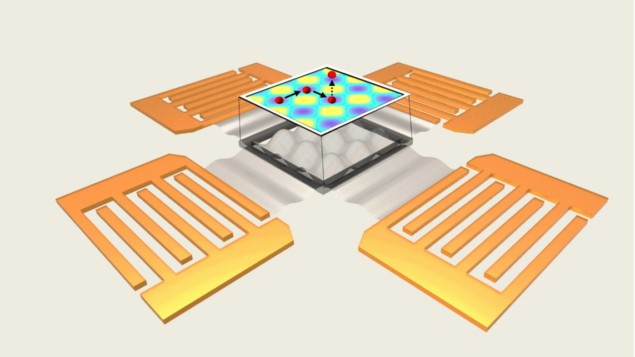
Will it ever be feasible to emulate Harry Potter’s levitation charm, “Wingardium Leviosa”, and manoeuvre tiny objects by scientific means? The manipulation of nanosized materials such as biological molecules, quantum dots and other inhomogeneous nanoparticles has been achieved via several approaches, including magnetic, electric and acoustic fields. The major drawback of many existing techniques, however, is their inability to control nano-objects over a large area. Furthermore, it is almost impossible to trap the tiniest particles and manipulate them over a large scale without creating additional background interference.
To overcome these limitations, scientists at Duke University developed a hybrid technology known as acoustoelectronic nanotweezers (AENT) to dynamically manipulate sub-100 nm particles. Instead of using sound waves to directly move the nanoparticles, AENT uses sound waves to create electric fields that provide the push.
AENT combines the benefits of electric tweezers and acoustic fields to precisely pattern the nanoparticles, while also controlling their orientation before they are eventually used for downstream applications – such as designing sensors to investigate disease, building devices for nanoelectronics and understanding particle–particle interactions.
In the past decade, the focus of nanomanipulation technology has been to capture nanomaterials and investigate their properties over a small area. However, with the AENT technology, scientists can now transport the trapped nanoparticles over larger distances and perform several tests at different positions and times. Most importantly, the researchers are able to trap particles smaller than 10 nm while minimizing the interference that often occurs in such devices due to acoustic streaming. Using this technology, it is possible to study DNA, proteins, exosomes and single nanoparticles at a high resolution.
Previous studies reported that combining acoustic and electric fields to trap particles could lead to excessive disturbances due to the forces acting on the nanomaterials. However, the team devised a means of minimizing this hydrodynamic interference by deploying integrated transducers on a piezoelectric (PZE) layer. This technique generates elastic deformations that propagate as acoustic waves through the PZE-layer and establish periodic electric fields.
‘’This method enabled us to perform levitation, transformation, particle pairing and rotation at a large [centimetre] scale with minimal hydrodynamic perturbations,’’ explains Tony Jun Huang, senior author of the study, details of which are published in Nature Communications.
To test the efficiency of the AENT technology, the researchers exposed polystyrene particles (100 nm) to acoustic waves in both electrically shielded and non-shielded regions. Less than 2 s after exposure, the particles were seen to form distinct linear patterns in the non-shielded region, while particles in the shielded region were seen to be stationary after their trapping.

The team also manipulated several other nanoscale objects including quantum dots (6 nm), exosomes (30–150 nm), silicon dioxide gold nanobeads (100 nm) and graphene flakes (30–40 nm), and observed similar patterns.
To demonstrate highly specific manipulation, the team managed to dynamically control single particles and push them around to spell out the letters D, U, K, E. The researchers believe that AENT holds great potential as a next-generation nanomanipulation technology that will find application across many disciplines, including nanofabrication, electronics, molecular physics, chemistry, metamaterials and biomedicine.
