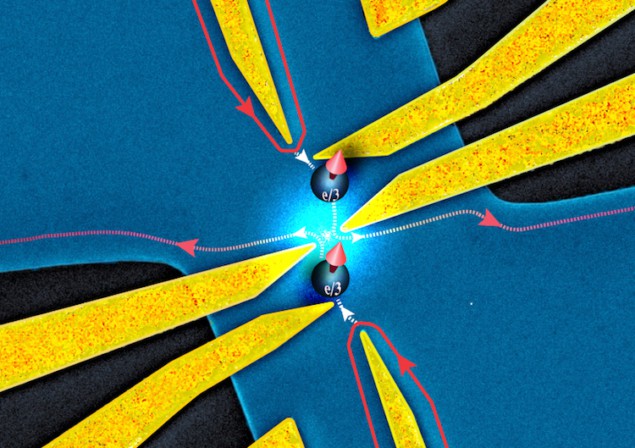
Anyons – the particle-like collective excitations that can exist in some 2D materials – tend to bunch together in a two-dimensional conductor. This behaviour, which has now been observed by physicists at the Laboratory of Physics of the ENS (LPENS) and the Center for Nanoscience and Nanotechnologies (C2N) in Paris, France, is completely different to that of electrons, and experimental evidence for it is important both for fundamental physics and for the potential future development of devices based on these exotic quasiparticles.
The everyday three-dimensional world contains two types of elementary particles: fermions and bosons. Fermions, such as electrons, obey the Pauli exclusion principle, meaning that no two fermions can ever occupy the same quantum state. This tendency to flee from each other is at the heart of a wide range of phenomena, including the electronic structure of atoms, the stability of neutron stars and the difference between metals (which conduct electric current) and insulators (which don’t). Bosons such as photons, on the other hand, tend to bunch together – a gregarious behaviour that gives rise to superfluid and superconducting behaviours when many bosons exist in the same quantum state.
Within the framework of quantum mechanics, fermions also differ from bosons in that they have antisymmetric wavefunctions – meaning that a minus sign (that is, a phase φ equal to π) is introduced whenever two fermions are exchanged. Bosons, in contrast, have symmetric wavefunctions that remain the same when two bosons are exchanged (φ=0).
Completely different situation in 2D
In two-dimensional systems, however, bosons and fermions are joined by other, more exotic types of elementary particles. In 2D electrical conductors, for example, the electrons interact very strongly with each other, and their collective movements can be viewed in terms of the motion of new elementary objects known as anyons.
The existence of anyons – which get their name from the fact that their behaviour is neither fermion-like or boson-like – was predicted in the early 1980s by the theoretical physicist Frank Wilczek. Soon afterwards, another physicist, Bert Halperin, found that anyons could explain certain aspects of the fractional quantum Hall effect, which describes the changes that take place in electronics at low temperatures in strong magnetic fields. Then, in 1984, Dan Arovas, Bob Schrieffer and Wilczek proved that a successful theory of the fractional quantum Hall effect does indeed require particles that are neither bosons or fermions.
As well having a different – fractional – charge from fermions or bosons, anyons are also predicted to obey different quantum statistics. To investigate these statistics, researchers have sought to devise experiments that can probe how the properties of an ensemble of particles are affected when two particles are exchanged.
Previous efforts have focused on directly measuring the phase that appears in the anyons’ wavefunctions when two of them are exchanged in an interferometer (a device that measures the phase difference between two waves). So far, however, LPENS team leader Gwendal Fève says that such experiments have proved tricky thanks to external effects that combine to modify the phase of the anyons’ wavefunction. These effects, known collectively as decoherence, tend to “wash out” quantum interference, and they are particularly strong in correlated systems.
Mini “anyon collider”
To overcome these problems, Fève and colleagues developed an anyon collision experiment that can measure the quantum statistics of particles and, in particular, determine whether the particles repel each other or bunch together. They began by using the weak coupling of electrons between two edges in a fractional quantum Hall fluid (in this case, a 2D electrical conductor made of an GaAs/AlGaAs electron gas) as a means of emitting anyons randomly – something that Fève says is not easy to do. They then collided the anyons on a beam splitter and measured the currents at its outputs.
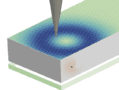
Anyons could be spotted using scanning tunnelling microscopy
The LPENS team created their miniature anyon collider using an electronic chip fabricated by their colleagues at C2N. To safeguard the anyons’ quantum properties, they kept the 2D electrical conductor on this chip at ultralow temperatures of 30 mK, and generated the collisions by applying a strong magnetic field (13 Tesla) perpendicular to the conductor.
In such a high magnetic field, all the electrons in the 2D electron gas will occupy the same energy level, and their interactions are very strong. This leads to the emergence of so-called “Abelian” anyons, which are predicted to appear when ν, the fraction of the energy level that is filled by electrons, is equal to 1/3, 1/5 and so on (that is, all fractions of 1/m, where m is an odd integer), Fève says. For ν=1/3, anyons are known to carry a fractional charge of e/3 (where e is the charge on the electron) and to obey fractional statistics. Their wavefunctions pick up a phase φ= π/3 when two anyons are exchanged, leading to behaviour that is intermediate between fermions and bosons.
Measuring current correlations
The researchers measured correlations in the electric current by measuring the currents at two outputs (labelled 1 and 2) of the beam splitter in their collision experiment. They amplified these currents – denoted by i1(t) and i2(t) – using custom-made cryogenic amplifiers since the signatures are very small. They then digitized them, computed the product of the two output currents, i1(t) x i2(t), and averaged this quantity over a large number of collisions.
“For uncorrelated currents, the average of the product i1(t) x i2(t), which we write < i1(t) i2(t)>, equals the product of the average currents, < i1(t)><i2(t)>,” explains Fève. “In the case of collisions between anyons, we measure negative correlations, meaning that <i1(t) i2(t)> is smaller than < i1(t)><i2(t)>.”
This negative correlation allowed the team to characterize the anyons’ tendency to regroup in packets of particles when they collide – a behaviour that demonstrates that they do not behave as electrons even though they originate from an ensemble of electrons, he says.

Majorana quasiparticles glimpsed in magnetic chains
“After a careful analysis and comparison with theoretical predictions for anyon collisions, we found that the degree of negative correlations we observe agrees well with the prediction that φ= π/3 for anyons at a filling factor ν=1/3,” he tells Physics World. This result corresponds to the quantum behaviour of anyons, thus demonstrating conclusively the existence of these exotic quasiparticles that are neither fermions nor bosons.
Non-Abelian anyons for topological quantum computing
For now, these findings are primarily of importance within fundamental science, says Fève, as they demonstrate that it is possible to manipulate new exotic particles with properties that differ from those of fermions and bosons. In the longer term, though, he suggests that the work could have implications for quantum computing. Although the anyons in this study were Abelian, in other (non-Abelian) anyons, exchange operations do not commute and thus cannot be described by a simple change in the phase of the wavefunction.
“In this case, exchanging particles could be used as elementary computing operations,” he explains. “A topological quantum computer making use of such operations would be much more robust compared to a conventional quantum computer (whose performance is currently strongly limited by fluctuations in the environment).”
The team, reporting its work in Science, says it would now like to investigate other filling factors corresponding to anyons with different quantum statistics. Now that recent experiments have shown that an energy-level filling factor of ν=5/2 can give rise to non-Abelian anyons, Fève says, “the filling ν=5/2 is one that we are looking at in particular”.