With NASA’s Juno mission to Jupiter arriving next week, researchers look to our local, ancient behemoth to figure out how planets form – including our own – as Stephen Ornes reports
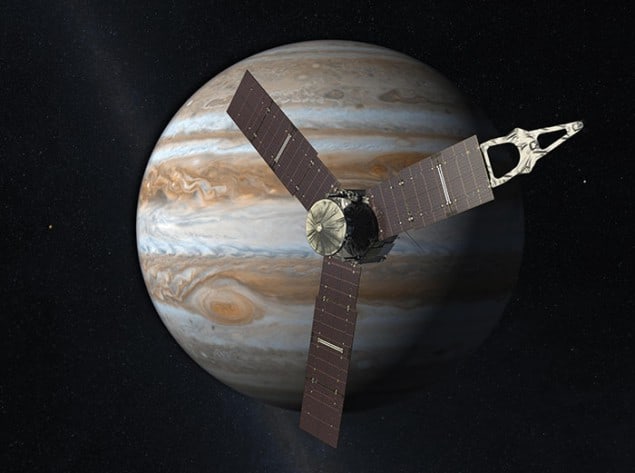
For the last five years, NASA’s Juno spacecraft has been barrelling towards its final destination: Jupiter, king of the planets. On 4 July this year (or 4 July in the US, at least, actually early morning on 5 July in Europe) the four-tonne, spinning craft – which looks like an oversized propeller that has abandoned its plane – will fire its thrusters and slow down enough to be captured by the gas giant’s gravity. The burn should only last about 40 minutes, but they’ll be a tense 40 minutes: during that time, as Juno shifts from orbiting the Sun to orbiting Jupiter, the rest of its devices will go quiet. (As will the physicists at NASA’s Jet Propulsion Laboratory in Pasadena, California, tracking the mission from the ground and, one imagines, with fingers crossed.) Then the instruments should flicker back on, and over the course of more than 35 long, loping polar orbits throughout the following year, Juno will execute an intimate and unprecedented observation of our local colossus, beaming data back to Earth.
Scott Bolton, Juno’s principal investigator, says the effort to study Jupiter is no less than a desire to understand the origin story of the solar system. Bolton is a space physicist at the Southwest Research Institute in San Antonio, Texas, and has led the $1.1bn mission from idea to execution. “When you want to understand where we all came from and how the planets were made, you have to start with Jupiter,” he says. Jupiter’s composition seems more star than planet, as it is dominated by hydrogen, followed by helium. At the same time, its atmosphere is drizzled with the heavier elements – including carbon, nitrogen and oxygen – that make life on Earth possible. “Jupiter is enriched with the same stuff we’re made of,” says Bolton. “We’re trying to understand our own history.”
Bolton’s words echo a growing wisdom among astronomers: if you want to know details about how the solar system formed, or how giant, gassy worlds coalesce around far-flung stars, you have to ask Jupiter. It likely formed early and fast, sweeping up most material left behind after the Sun formed. Jupiter is more than twice as massive as all the other planets, moons, asteroids, comets and Kuiper-belt objects in our system combined. Because of its age and heft, the gas giant probably played a critical role in arranging the solar system, helping to jockey planets into their current positions. (With 67 known moons, it effectively hosts its own planetary system too.) Astronomers even credit Jupiter’s gravitational oomph for diverting comets and asteroids that might otherwise have plummeted into Earth and brought a quick end to life as we know it.
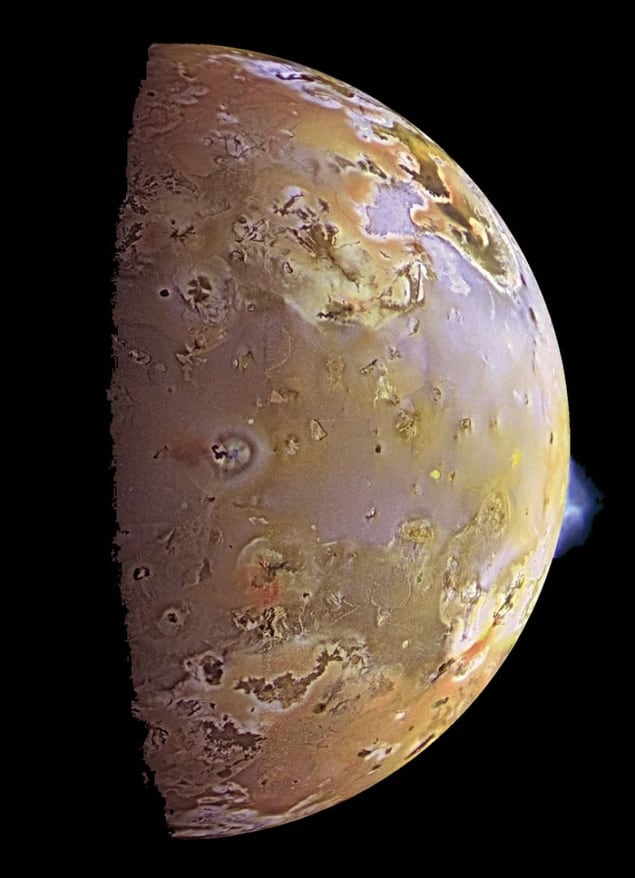
Ancient civilizations watched the planet with awe and interest, and astronomers have been probing its mysteries since Galileo Galilei first studied it and its moons through a telescope more than 400 years ago – observations that showed that not everything in the heavens orbits Earth. The more scientists learn about Jupiter, the more unknowns they find. Even after centuries of inquiry, Jupiter is shrouded in mystery.
Scientists don’t know the structure of its thick, hot atmosphere, or how much water that atmosphere contains. Even more mysterious is the structure of its centre, hidden far below. Brilliant light shows, called auroras, encircle the opposite poles like twin crowns – and even exist deeper in the atmosphere – though researchers disagree on how they form (see “Extraterrestrial light shows”, July 2016, pp37–39 in print). Ground-based observations of Jupiter and its moons offer tantalizing hints at answers to these mysteries, but the best way to ask how the king of planets ticks is to go there and see for ourselves.
Juno’s goals are simple. How did the planet form and evolve? What hides beneath Jupiter’s clouds? The answers to those puzzles may help answer even bigger questions about why planets form at all. “We’re after the recipe for the solar system,” Bolton says.
The story so far
In Roman mythology, Juno was the wife (and sister) of Jupiter, king of the gods, and she didn’t take kindly to his extramarital interests. To conceal an affair with a mortal priestess named Io, Jupiter concealed himself with a dense cloud cover. Not to be fooled, Juno handily swept the clouds aside – an action that resonates with the modern Juno mission.
The planet Jupiter, long a source of fascination for stargazers, appears in one of the first bona fide science-fiction stories. In 1752 French philosopher Voltaire published Micromégas, a story that reads a bit like Gulliver’s Travels, but in space. The tale follows the adventures of a 37 km-tall alien and his 2 km-tall friend as they compare experiences and explore the solar system. Their trajectory takes them to the moons of Jupiter and briefly to the planet itself: “They stopped at Jupiter and stayed for a week, during which time they learned some very wonderful secrets,” which are not, unfortunately, revealed in the story. (The duo later visits Earth, but as its inhabitants are too small to be seen, they dismiss the possibility of finding intelligent life there.)
The Juno mission represents the ninth Jovian visit by a human-built ship. The most recent was New Horizons, en route to Pluto, in 2007. The first arrived in 1972, when the Pioneer 10 spacecraft snapped 300 images and took measurements as it zoomed by at 132,000 km/h, about 130,000 km above the tops of the clouds. Data from that mission helped scientists to make early hypotheses about the fluid-filled interior and to analyse plasma in the planet’s giant magnetosphere – the region in which charged particles are affected by Jupiter’s magnetic field. The mission wasn’t entirely smooth sailing: some of the onboard instruments malfunctioned due to the intensity of the radiation surrounding Jupiter, but those problems helped guide the design of better protection for future missions. The next year, on its way to Saturn, Pioneer 11 flew by Jupiter, even lower and faster than its predecessor. The Voyager 1 and 2 missions followed in the late 1970s, sending back more data – and unanswered questions.
“The Voyager missions opened up a bunch of unknowns,” says physicist Theodor Kostiuk of NASA’s Goddard Space Flight Center in Maryland, who uses ground-based telescopes to study Jupiter’s atmosphere. Voyager, for example, identified active volcanoes on Io, a large inner moon, that affect the entire planetary system. (Prior to Voyager, astronomers didn’t know that volcanic activity existed anywhere else in the universe.)

The Ulysses spacecraft measured Jupiter’s magnetosphere during flybys in 1992 and 2000, when it used gravitational assists from the planet to slingshot itself towards the Sun, its primary research target. The Cassini–Huygens spacecraft, while en route to Saturn, took tens of thousands of pictures of Jupiter and made detailed measurements of its atmosphere during a six-month period in 2000 and 2001. The first orbiter to reach Jupiter was Galileo, which spent eight years circling the planet’s equator and studying the Jovian moons, but it ran into problems and did not ultimately fulfil all of its scientific goals.
Planetary space physicist Fran Bagenal, of the University of Colorado at Boulder, worked on Galileo’s science team and now leads the plasma research teams for both Juno and New Horizons, the mission that reached Pluto last year (see “Our new view of Pluto”, July 2016, pp40–43 in print). “Galileo’s observations told us a lot about the moons, but it had this problem,” she says. In 1991 Galileo’s 4.8 m high-gain antenna, which was shaped like an umbrella and designed to radio data back to Earth, only partially opened. Scientists tried for five years to fix the problem from Earth, but to no avail. “It meant we couldn’t do a lot at the planet itself,” Bagenal recalls. The mission couldn’t send back as much data as scientists had anticipated, and the spacecraft disintegrated during its intentional, final plunge into Jupiter’s turbulent atmosphere.
Juno is the scientific heir to Galileo, but it differs in important ways. Galileo’s price tag was about $1.4bn, whereas Juno’s estimated cost is about $1.1bn. Where Galileo circumnavigated the equator, Juno will orbit the poles. Galileo, like most spacecraft, used nuclear fuel to travel through space. Juno relies on solar power. Its three radial arms are 9 m arrays that hold 19,000 solar cells, and in January of this year Juno set a record for the farthest distance travelled using solar power. (The record was previously held by the European Space Agency’s Rosetta spacecraft, which travelled to the asteroid belt between Mars and Jupiter.)
In Earth’s neighbourhood, Juno’s solar cells receive enough sunlight to generate 14 kW – which could power 10 microwave ovens at once. Near Jupiter, where sunlight is weaker, the cells collect only enough light for about 400 W. That’s not enough to power a hair dryer, but it’s sufficient for Juno’s suite of scientific instruments. “It demonstrates that solar power works in a new environment that we hadn’t thought possible,” says Bolton. The radiation belts around Jupiter, he says, are “one of the harshest regions in the solar system”.
Preparing for the storm
Jupiter is notoriously inhospitable. Winds blow at 650 km/h or more. Lightning strikes with 100 times the intensity of lightning on Earth. The Great Red Spot – the solar system’s biggest storm, which has been raging for more than three centuries – is so big it could swallow Venus.
The planet’s biggest threat to space travel, though, is radiation. Jupiter’s magnetic field is 10 times stronger than Earth’s. Indeed, its magnetosphere is the largest known structure in the entire solar system. If it glowed visibly, the magnetosphere would appear to observers on Earth more than twice as big as the full Moon. Such a sprawling magnetosphere traps a lot of high-energy particles, creating radiation belts that circle Jupiter, forming what must be the most hazardous doughnut in space. (The belts are similar in shape and structure to Earth’s Van Allen belts.)
“You’ve got this stream of electrons and protons circling the planet, and they’re lethal to spacecraft,” says astronomer and Juno team member Tobias Owen of the University of Hawaii, whose goal is to measure oxygen in Jupiter’s atmosphere. “Until now, spacecraft have been farther out. We’re going to be inside it.”

Instruments onboard Juno include a particle detector, magnetometer, ultraviolet and infrared spectrometers, and radio instruments for measuring fluctuations in the gravitational field. (The payload also includes three LEGO figurines, representing Juno, Jupiter and Galileo.) The electronic devices would ordinarily be crippled by the intense radiation, which is why they are safely housed in a protective vault with centimetre-thick titanium walls. Juno’s flight plan, which takes it over the poles, will also reduce exposure to the powerful radiation.
The same magnetic field that makes the mission so treacherous embodies one of the planet’s most pressing mysteries. Io’s active volcanoes, as Voyager observed, spew sulphur-dioxide particles that become ionized and fill the magnetosphere, says Bagenal. As they accelerate to high energies, many of the particles end up bombarding the atmosphere of Jupiter – a process that’s believed to contribute to the auroral light shows at the poles.
However, “we’ve never flown over the poles of Jupiter before, and we don’t know what it’s like up there” says Bagenal, whose research focuses on plasma in planetary magnetospheres. “We don’t know what processes accelerate those particles into the atmosphere.” Juno, she says, will be able to measure the magnetic field, charged particles and plasma waves as it looks down on the auroral emissions in the atmosphere. “We’re trying to put together the bigger picture of what causes the auroras, and how they work.”
Beneath the clouds
It’s tempting to assume that Jupiter’s auroras form like those on Earth, which arise after charged particles from the solar wind are accelerated along magnetic field lines into the upper atmosphere, where they collide with other particles and emit light. But such an explanation might be too simplistic for Jupiter. Ultraviolet images taken by the Hubble Space Telescope 20 years ago show that the auroras form round, oval-shaped structures near the poles. Though small-scale changes occasionally occur within and to each oval, “it really doesn’t vary a whole lot” says Bagenal.
That might be in part because Jupiter’s powerful magnetosphere protects its atmosphere from the solar wind. In that case, the auroras might be generated internally, from “an atmospheric region deep in the atmosphere” says Kostiuk. Using infrared imaging, Voyager identified a thermal aurora deeper in the atmosphere in the north hemisphere, which researchers have studied for three decades with ground-based measurements and, in 2001, data from the Cassini flyby. Kostiuk points out that recent ground-based measurements of the aurora in the infrared do show some variation with the solar cycle – suggesting a contribution from the solar wind.
From within or without? “That’s the big debate,” says Bagenal. She suspects the auroral emissions arise from how the plasma in the magnetosphere moves with respect to the planet, which completes a rotation in just under 10 hours. “At some point, the clutch begins to slip,” she says. “We think electric currents associated with that process are partly driving the auroras.” But scientists won’t know for certain until Juno takes a look.
Another of Juno’s scientific goals is to better understand the colourful, swirling bands of clouds. To date, it’s been difficult for scientists to probe the depths because it’s too hot, and the pressure is too great. Juno’s multi-frequency microwave radiometer will receive thermal radiation from the depths of Jupiter’s cloud cover, up to pressures about 1000 times Earth’s normal atmospheric pressure at sea level. That penetration will help researchers better understand the rotation of the atmosphere relative to the core – if it exists – and what elements exist there.

“The thing that’s most exciting to me is the determination of water deep in the atmosphere,” says Owen. “By measuring the water we’ll get an idea of the way that Jupiter came together.” Many astronomers have proposed models to explain Jupiter’s formation, but different models predict different levels of water. Measuring that abundance, says Owen, will help models get closer to approximating the origins of the planet. “Water abundance is key if you’re trying to understand how planets are formed in our solar system,” says Bolton.
Juno will also be studying what lies beneath the clouds. The planet likely contains a vast and bizarre ocean unlike anything found on Earth – and unlike anything that can even be simulated on Earth. It’s made of hydrogen under so much pressure that electrons separate from protons, and the fluid conducts electricity like a metal. As this strange sea rotates with the planet, it generates Jupiter’s powerful magnetic field. “We think that’s where the dynamo is produced,” says Bagenal.
But scientists don’t know how deep the liquid metallic hydrogen extends, or what’s underneath it. They hypothesize that Jupiter’s core is rocky and made of heavier elements. Since no device could reach the hydrogen sea – much less any core that lies beneath – Juno will map the interior structure by tracking changes in the planet’s gravitational field as it orbits.
The end of Juno
Juno will spend a full year measuring and sending data to astrophysicists on Earth, but Bolton warns that definite answers about Jupiter won’t show up immediately. “We’re limited on how we can interpret Juno’s data,” he says. Using gravity measurements to map the distribution of mass will be fairly straightforward. But to connect data on variables like temperature and pressure and forge one big, coherent picture will require physicists to agree on an equation of state to describe the conditions on Jupiter. That’s a challenge in and of itself: no-one has any idea how metallic hydrogen is supposed to behave. They also suspect, but can’t prove, that heavier elements likely dissolve in that strange soup.
Bagenal says the equation of state is a crucial and missing piece of the puzzle. “Every time we have a meeting of the interior working group of the Juno mission, these guys come up with a new equation of state,” she says. “They’re always improving, and always changing their minds. Since we launched, they’ve changed their minds a few times.”
At the same time, Juno’s data will immediately be put to use by theorists who come up with models of how Jupiter formed. “All theories on how Jupiter forms will have to be consistent with what Juno sees,” says Bolton. “By making these measurements, we will constrain the models.” Those limitations will, in turn, lead to more refined models that more accurately represent the reality of Jupiter.
Once Juno’s year-long data-gathering feast is over, it will change direction one last time. The spacecraft won’t be allowed to orbit indefinitely because of the unlikely chance it might collide with and contaminate Europa, a Jovian moon with a subsurface ocean where, one day, scientists would like to look for life. So instead of drifting off, Juno will end, like Galileo before it, by disintegrating during a final plunge into the heart of its host.