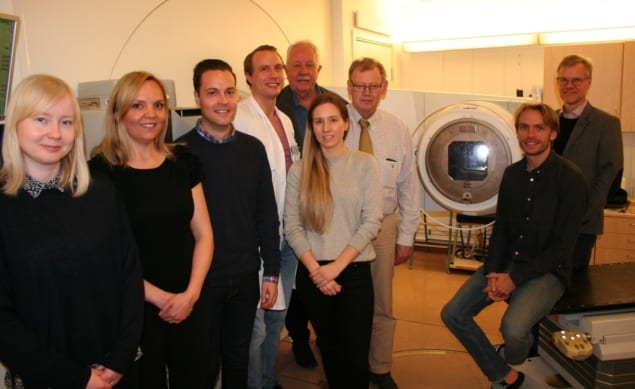
FLASH radiotherapy (FLASH-RT), the delivery of ultrahigh radiation doses in fractions of a second, is under investigation as a cutting-edge technology to improve cancer treatment. Research and pre-clinical studies have been limited, however, by the need to use highly specialized equipment.
Medical physicists and biomedical engineers in Sweden have now developed a way to modify a conventional linear accelerator for FLASH irradiation — and to rapidly restore it for clinical use without interfering with cancer patient treatment schedules. Such use of clinical linacs could increase the pace of global research into FLASH-RT (Radiother. Oncol. 10.1016/j.radonc.2019.01.031).
Developed by Vincent Favaudon, a radiobiologist at Institut Curie, FLASH radiation delivery employs dose rates of 30 to more than 106 Gy/s, at least a few hundred times higher than conventionally used in radiotherapy. The potential advantages are significant.
An ultrahigh radiation dose delivered in milliseconds could provide more effective tumour treatment. The impact of patient motion during irradiation would be significantly minimized with this rapid delivery, reducing the need for target margins and thereby the volume of healthy tissue being irradiated. With fewer treatments, the problem of inter-fraction motion could be minimized or eliminated. Fewer and faster treatments will also allow radiotherapy treatment rooms to accommodate more patients, significantly expanding their utilization.
Linac testing
Principal investigator Kristoffer Petersson, and colleagues from Skåne University Hospital and Lund University, modified a clinical linac (ELEKTA Precise), connecting it to an in-house developed electrical circuit for pulse-to-pulse control. They controlled the radiation delivery using a diode as an electron beam pulse radiation detector: once the programmed amount of beam pulses had been delivered, the beam was interrupted. The researchers used three set-up positions with reduced distance from the target. They adjusted and optimized gun current, modulator charge rate, and beam steering values to produce a high dose rate.
The linac reached a FLASH irradiation dose rate at the cross-hair foil, multileaf collimator and wedge positions, with dose rates of at least 30, 80 and 300 Gy/s, respectively. Moving the scattering foils from the beam path increased the output to at least 120, 250 and 1000 Gy/s at the three positions.
The beam flatness was 5% at the cross-hair position for a 20 x 20 and a 10 x 10 cm area, with and without scattering foils in the beam path. Beam flatness was 10% at the wedge position for a 6 and 2.5 cm diametric area, with and without the scattering foils. The researchers have subsequently been able to increase the dose rate by a factor of four.
The researchers note that the modified linac had some stability issues. The output was high and relatively stable during the first 10 minutes following a warm-up procedure, but subsequently decreased, requiring a new warm-up to restore stability. The researchers have since resolved these stability issues, achieving a standard output deviation of around 2%, which is acceptable for pre-clinical studies.
The authors recommend that the FLASH beam should be monitored on a dose level, and not just on a pulse level, to achieve better control over the delivered radiation dose and to assess pulse-to-pulse variations.
“One way of doing this is by slightly varying the gun filament current setting and thereby the pulse amplitude, so that the delivery of a fixed number of pulses would result in the delivery of a desired dose,” Petersson tells Physics World. “We have recently tested this method and it works fine. However, the gun current value resulting in a specific pulse amplitude varies slightly from one day to another due to the wear of the filament, and this needs to be taken into account for the method to be precise.”
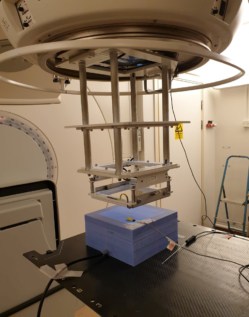
Petersson adds that a real-time dose monitoring device would provide more sophisticated dose monitoring. “We have been able to extract the signal from the transmission chamber and are currently studying how the ion collection efficiency of the transmission chamber drops with increasing dose-per-pulse values in the beam, which, if correctly modelled, would give us this device,” he explains. “The second step would be to use the information to modify the length or amplitude of the pulses on a pulse-by-pulse basis, to ensure delivery of the desired dose.” The researchers have not yet tested this second step as it would require major linac modifications.
The team is currently performing cell studies with the FLASH beam. Using cells prepared at different oxygen concentrations, they aim to determine whether the FLASH effect is due to oxygen consumption and the delivery being too quick for oxygen levels to replenish during the delivery. This would result in tissues behaving as if they were hypoxic.
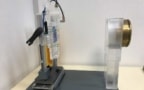
FLASH proton delivery aims to reduce radiotherapy toxicity
“We are planning additional studies with the team of Kevin Prise at Queen’s University Belfast, to look at bystander effects and how these vary with dose rate,” Petersson says. “We are also preparing studies on zebrafish embryos to investigate the induction of malformation and how this depends on dose rate, in conjunction with radiobiologist Marie-Catherine Vozenin at Lausanne University Hospital.”
FLASH-RT may be ready for testing in humans in three to five years. Before clinical trials can be safely performed, researchers need to improve dosimetry in beams with ultrahigh dose rates, develop a dose delivery control system, and better understand the underlying mechanisms responsible for the FLASH effect, such as oxygen consumption.