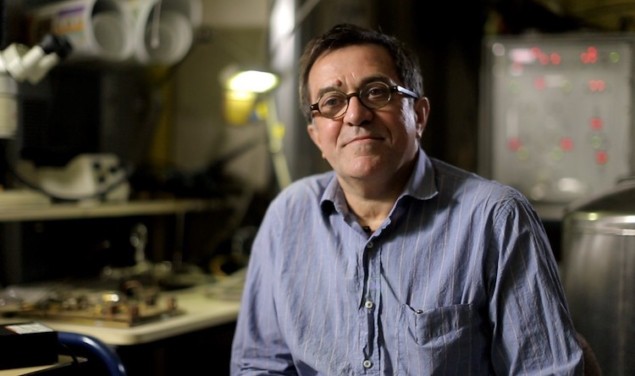
The quantum Hall effect (QHE) is one of the most important effects being studied by solid-state physicists today. Measuring the limits at which it breaks down is extremely important – not only for fundamental physics but also for applying the effect as a resistance standard for redefining the kilogram. Researchers in France have now found that collective excitations of interacting electrons are responsible for the onset of the breakdown of the QHE in bilayer graphene at high electric fields and they have even calculated the “Landau velocity” at which this happens. The new result lends weight to the idea that the long-held single-electron picture is not a realistic description of the QHE. The breakdown mechanism also looks very much like what happens in superconductors at the limit at which correlated electron pairs (responsible for the supercurrent in these materials) break apart and at the point at which superfluidity collapses in systems like liquid helium.
Universal value for the quantum Hall resistance
The quantum Hall effect (QHE) is the appearance of a voltage across opposite faces of a thin, 2D conducting sheet (like graphene, which is a layer of carbon just one atom thick) when a current is passed along the plane of the sheet and a magnetic field is applied perpendicular to it. The magnetic field forces conduction electrons in the sheet to drift in circular, quantized, orbits (known as Landau levels). This movement normally depends on factors such as the density of electrons in the material and the thickness of the sheet. When the Hall voltage is compared with the current running through the sheet, the resulting quantum Hall resistance is h/Ne2, where h is the Planck constant (h), e is the electron charge and N is an integer.
All experiments to date appear to agree on a universal value for the quantum Hall resistance. “The accuracy of the experimental determination of this resistance may rely on correlated particle effects like those we have observed in our study though,” says Bernard Plaçais of the Laboratoire Pierre Aigrain in the Physics Department at the Ecole Normale Supérieure in Paris, who led this research effort. “Besides, graphene, which has a high electron mobility and in which the quantum Hall resistance has been measured with great accuracy, is now recognized as being a valuable platform for making a future QHE resistance standard.”
At the moment, the kilogram is defined by a small platinum and iridium cylinder crafted in 1889 and held at the Bureau International des Poids et Mesures in Paris, but recent comparisons of this standard with identical “witness” copies suggest that its mass is changing. Researchers would thus like to redefine the kilogram in a new way based on fundamental constants alone and one promising way to do this is in terms of the quantum Hall resistance.
Collective excitations of interacting electrons
Thanks to high-frequency shot-noise measurements, which were employed for the first time in this context, Plaçais and colleagues have now found that, at high electric fields, the QHE in bilayer graphene samples breaks down at a critical electron drift velocity thanks to collective excitations of electrons with a large momentum at the so-called magneto-exciton minimum. “This result is exciting because until now the QHE had been described by a single electron picture,” explains Plaçais. “We show here that this picture is incorrect at high electric fields where correlated electron transport effects are at play.
This situation is very much reminiscent of the superconducting currents in a superconductor, he states. “These currents are protected by the superconducting gap up to the limit at which the superconducting electron pairs (responsible for the supercurrent in these materials) break apart.
Analogies with the breakdown of superfluidity
“We studied the critical field at which this Hall insulator to metal transition occurs n collaboration with our theory colleagues from LPS-Orsay (led by Mark Goerbig) and ENS Lyon (led by David Carpentier),” he continues. The explanation we put forward also closely matches the breakdown of superfluidity in liquid helium. Helium gas is a very weakly interacting system, but it can be liquefied by applying a large pressure and transforms into a cloud of liquid helium droplets (in the so-called Joule-Thomson transformation).
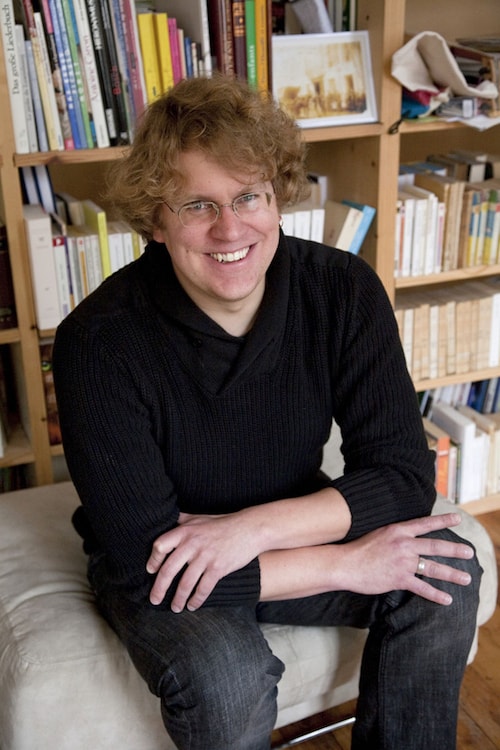
“In our system, the high electric field is analogous to the large pressure, and collective excitations of the Hall insulators (the magneto-excitons) analogous to the liquid helium droplets,” he explains. “These magneto-excitons disperse in a particular way thanks to the short-range interactions between them. This dispersion drastically reduces the excitation energy at finite wavelengths (the Landau gap), which extends to around the same length as the inverse of the so-called inter-Landau Level (LL) wavefunction distance.
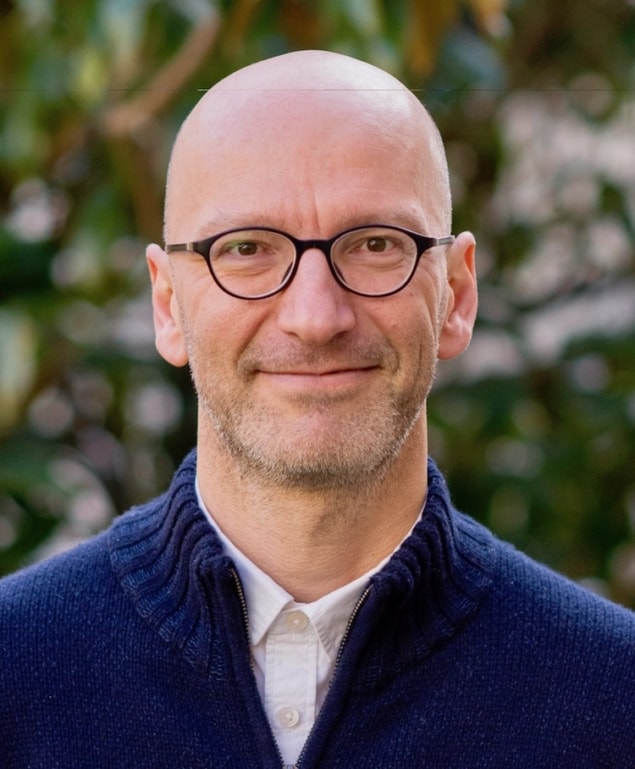
“When electrons in bilayer graphene are submitted to a high electric field, their dispersion is simply modified as a Galilean transformation resulting from their drift velocity. The Landau gap can then reach zero and even become negative. This phenomenon is known as the critical Landau velocity and is responsible for the proliferation of collective electronic excitations dissipating the current.”
Until now, researchers believed that single electron tunnelling in 2D materials like graphene started from the last occupied LL and gradually propagated to the deeper-lying LLs. “Our experiments invalidate this picture,” Plaçais tells Physics World. “The scenario we put forward is completely different in that the electron excitations are collective and involve the full set of occupied and unoccupied LLs. This is why it occurs so suddenly and looks like a bulk phase transition.”
The work is detailed in Physical Review Letters 10.1103/PhysRevLett.121.136804.