Born at the dawn of time, primordial black holes may lurk throughout the universe – should they exist. But what if one struck the Earth or even, perhaps, a human being? Ian Randall discovers whether there is legitimate cause for concern or if such a scenario is mere science fiction
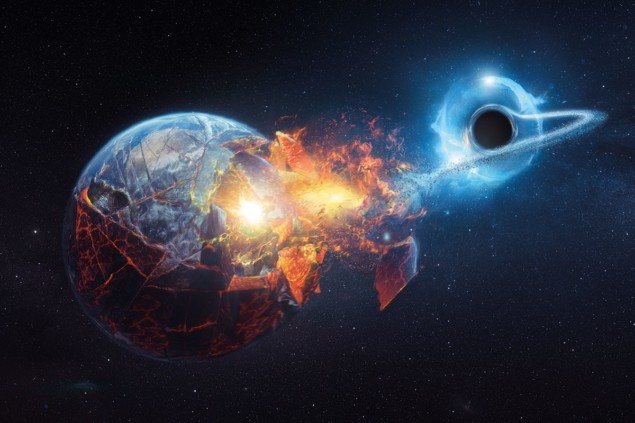
It’s just gone 12:45 p.m. on 30 November 1954 when a lazy Alabama afternoon is suddenly arrested by a fireball noisily rending the air, burning so bright it’s visible from two neighbouring states as it streaks through the sky above Sylacauga. Breaking into fragments, one grapefruit-sized part of the rock slams messily through the roof of a farmhouse, ricochets off a large console radio and slams into the side of a young woman as she takes a nap on her couch. It was thus that the unfortunate Ann Elizabeth Fowler Hodges entered the annals of history as the first individual known with certainty to have not only been struck by a meteorite but also to have lived, if severely bruised, to tell the tale.
Perhaps it is a morbid curiosity innate to the human condition, but the prospect of death and destruction raining down from the heavens has long fascinated us. Poor Ann Hodges was met by some 200 reporters outside her house following her ordeal, while the dinosaur-killing Chicxulub asteroid makes tabloid headlines on a monthly basis, despite having impacted some 66 million years ago. It is no surprise, then, that as cosmologists delve into the prospect that our universe may be rich in tiny black holes, one scientist has turned his hand to calculating the likelihood and risks of one colliding with the Earth.
More holes than Swiss cheese
The idea that black holes might have formed soon after the Big Bang dates back to 1966, when their existence was first proposed by physicists Yakov Borisovich Zel’dovich and Igor Dmitriyevich Novikov. By the mid-1970s the concept was picked up and developed by Stephen Hawking and his colleague Bernard Carr.
Our current theory for how the universe formed does not allow for black holes being created in the early universe. Instead, stars and galaxies first formed thanks to fluctuations in the early universe. Black holes formed much later, only after the first ancient stars had died.
But if primordial black holes (PBHs) did form as early as one second after the Big Bang, during the “radiation dominated” era, things would be different. Hawking and Carr argued that PBHs could have formed as the result of the same density fluctuations in the early universe that we currently believe created the first stars and galaxies. In their model, however, regions of the universe with slightly more mass than average could have collapsed in on themselves to form these embryonic black holes. “The existence of galaxies today implies that the early universe must have been inhomogeneous,” the duo wrote in a 1974 paper (MNRAS 168 399). “Some regions might have got so compressed that they underwent gravitational collapse to produce black holes.”
Hawking and Carr predicted that PBHs could have formed with a wide range of possible sizes, from as small as 10 μg to as large as 10,000 solar masses. This is quite unlike their stellar counterparts, which tend to be between 5 and 10 solar masses and are constrained by the size of the star that collapsed to form them (see box at end of feature).
As early as 1975, other physicists began raising an intriguing possibility about these early black holes. As Hawking had first realized, black holes evaporate over time, via Hawking radiation. But what about PBHs larger than 1011 kg (about the size of a small asteroid) that had not totally evaporated? Might they provide a neat explanation for part or all of the missing dark matter needed to account for the discrepancy between the cosmos’ visible matter and the observed motion of the stars and galaxies? The idea has faded in and out of vogue over the decades and, as Carr himself noted in 2020, “As with other cold dark matter candidates, there is still no compelling evidence that primordial black holes provide the dark matter.”

Particular interest arose in the 1990s – following the development of the theory of cosmic inflation, which finally provided an explanation as to where the universe’s original density fluctuations might have come from. The notion also attracted more recent interest following the first detection by LIGO–Virgo in 2016 of the gravitational waves caused by a binary black hole merger. The scale of the holes in question – in the range of 10–50 solar masses – was larger than had been expected, leading some to speculate the pair were primordial in origin.
Since then, LIGO has detected dozens more black-hole mergers (figure 1). And according to a Bayesian analysis published last year by cosmologist Gabriele Franciolini of the University of Geneva and his colleagues (arXiv:2105.03349), as many of as 24 of these binary black holes could well have been formed in the early universe.
A long time ago
The idea that PBHs could truly be the source of dark matter in the universe received a further boost this year, thanks to a study by Yale University researcher Priyamvada Natarajan and colleagues (ApJ 926 205). They propose that if the lion’s share of PBHs were formed with a mass around 1.4 times that of the Sun, then they could account for all dark matter in the universe. The Yale team’s model suggests that these PBHs could have provided the gravitational anchors around which the first stars and galaxies formed. Indeed, these early black holes could, according to the team, have consumed the stars and gas in their vicinity, making them the predecessors of the supermassive black holes that sit at the heart of galaxies today.
“Primordial black holes, if they do exist, could well be the seeds from which all supermassive black holes form, including the one at the centre of the Milky Way,” says Natarajan. “What I find personally super exciting about this idea is how it elegantly unifies the two really challenging problems that I work on – that of probing the nature of dark matter, and the formation and growth of black holes – and resolves them in one fell swoop.”
The existence of PBHs may also resolve another long-standing cosmological puzzle – namely that of the excess of infrared radiation that astronomers have detected coming from various dim, distant sources across the universe. According to Natarajan and her team, their model perfectly predicts that the growth of PBHs – thanks to the accretion of matter – would produce exactly the same radiation signature.
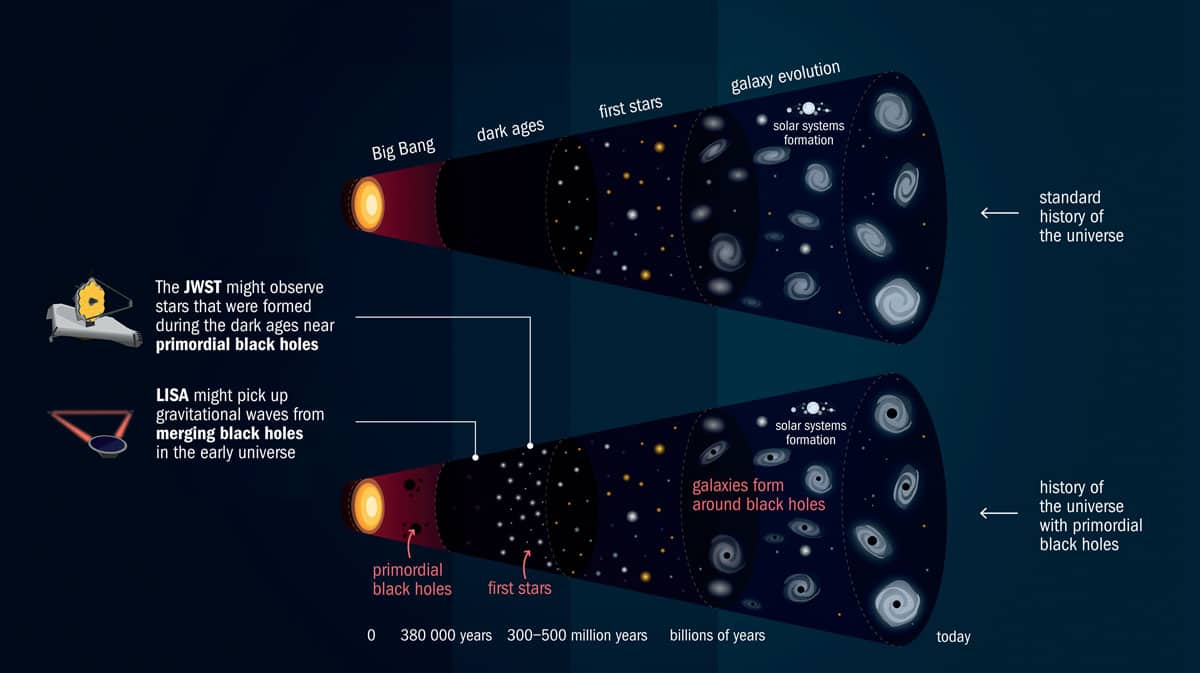
The good news is that the existence of PBHs in the early universe is something that we should be able to either confirm or rule out in the near future. That’s because one of the main aims of the James Webb Space Telescope (JWST) is to detect the first galaxies and stars to form in the distant universe. Like a Christmas present from NASA, the scope was launched on 25 December 2021 and is currently being commissioned, with its 18 primary mirror segments having just been aligned.
“If the first stars and galaxies already formed in the so-called ‘dark ages’, the JWST should be able to see evidence of them,” says Natarajan’s colleague Günther Hasinger, director of science at the European Space Agency (ESA). The telescope should also be easily able to detect the deep infrared sources in the distant universe, as suggested in the Yale model (figure 2).
In around a decade and a half, meanwhile, ESA expects to launch the three spacecraft that will make up the Laser Interferometer Space Antenna (LISA) gravitational wave detector. If PBHs existed and merged in the early universe, they should have produced characteristic gravitational wave signals that LISA will be able to detect.
Closer to home
So given that PBHs may well abound in the universe, just how likely is it that one might impact the Earth, and what would happen if it did?
Working on the assumption that PBHs account for all the dark matter in the Milky Way’s galactic halo and dark disc, Sohrab Rahvar from Sharif University in Iran has calculated that we might expect the Earth to collide with a small PBH – one with a mass of 1012 kg – around once every billion years (MNRAS 507 914). This, he explains, is at the lower range of PBH sizes that could have survived evaporation by Hawking radiation over the current lifetime of the universe (any black hole born soon after the Big Bang with a mass less than 1011 kg will have evaporated by now). While larger holes may still exist, the rate at which they would collide with Earth is less than one collision across the lifetime of our planet.
“In this scenario, the Earth is moving through the dark halo and can attract primordial black holes,” says Rahvar. As he notes, such a PBH would pass through the Earth, as its kinetic energy is too high to be trapped within. The PBH would be slowed down by a combination of dynamic friction from gravitational interactions with the material of the planet, as well as momentum transfer from the accretion of material onto the black hole.
From this, one can imagine two potential outcomes from a black hole colliding with Earth. One possibility is that it would pass through and carry on out the other side, transferring some of its energy in the process. Alternatively, the black hole would be slowed down so much that it spirals down to the planet’s core. At this point, Rahvar explains, the Earth would be doomed. “The accretion of matter into the black hole will heat the interior of the Earth and make the black hole grow – and finally, all the Earth will be swallowed by the black hole.”
Fortunately for our general existence, his calculations indicate that if PBHs do make up the dark-matter structure of our galaxy, they would have such a high dispersion velocity – in the order of 200 km/s – that they could only make a flying visit through our home, rather than outstaying their welcome. “We would expect there might be up to four primordial black hole collisions during the lifetime of the Earth,” Rahvar says, adding that “even in the case of collision, fortunately, the chance of trapping one inside the Earth is almost zero.”
Clash of the space killers
Even if a PBH isn’t going to destroy the Earth, we might still wonder what happens when one whizzes through our planet, and whether it might leave any traces that we could detect as a proxy for their existence. With this in mind, Rahvar calculated that the impact of a hypothetical asteroid would actually release five times more energy than that of a PBH of equal mass. And even if they were equal, black holes would be far less Armageddon-inducing than an asteroid.
The reason for this, he explains, is that asteroids release their energy in the atmosphere and surface of the Earth by an explosion, but primordial black holes produce heat by passing through the interior of the Earth. The heat released by a black hole’s transit through the planet would be “less than 1015 joules”, he adds, which is “small compared to the internal energy of the Earth”.
Nevertheless, a PBH would have a notable local impact, Rahvar notes. “The passage of a black hole could melt a cylinder along the interior of the Earth with a radius of almost 10 cm. After a short time, this tunnel would freeze,” he explains. In theory, this would produce a distinct metamorphic fingerprint of the passage in the rock record. But given that we might expect only eight of these to have been produced in the Earth’s lifetime (one on each side of the planet per collision episode), “I would expect that such a geological trace is difficult to detect,” Rahvar concedes.
All about that mass
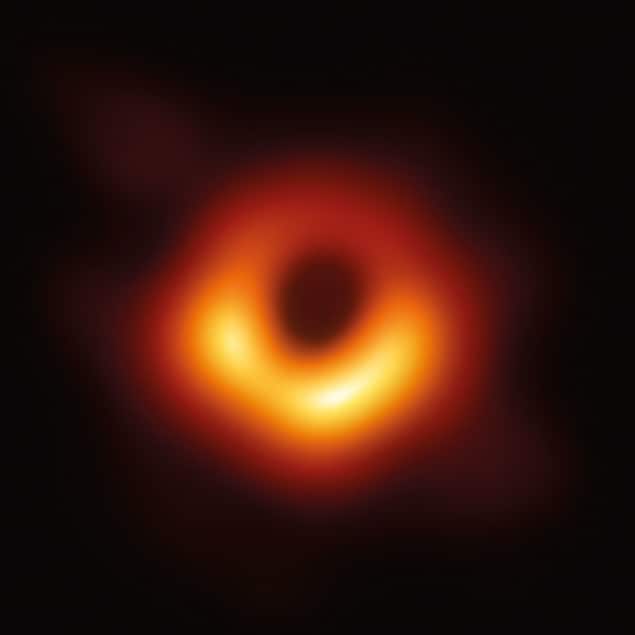
Stellar-mass black holes
Created by the gravitational collapse of massive stars at the end of their lives, these black holes are usually between 5 and several 10s of solar masses.
Intermediate-mass black hole (IMBH)
Weighing in at significantly more than a stellar black hole, these mid-range objects have a mass of 102–105 solar masses. Several IMBH candidate objects have been discovered in our galaxy and others nearby. Most prominently, the gravitational-wave signal GW190521 detected by LIGO on 21 May 2019 resulted from the merger of two black holes, weighing 85 and 65 solar masses, with the resulting black hole coming in at 142 solar masses.
Supermassive black hole (SMBH)
As the largest type of black hole (and indeed one of the largest structures in our universe) supermassive black holes – those with millions to billions of solar masses – most likely sit at the heart of every large galaxy. Our own Milky Way hosts the Sagittarius A* supermassive black hole in its galactic centre. The only black hole ever to have been imaged – by the Event Horizon Telescope in 2019 – is the behemoth that lies at the heart of the nearby M87 galaxy. This SMBH has a mass 6.5 billion times that of the Sun.
Infinitesimal chance, deathly outcomes
The chances of Earth, let alone a person, being hit by a black hole in our lifetime may be infinitesimal – but, as the popular meme goes, it is never zero. So, then, what would actually happen if you were struck by a primordial black hole? Researchers have suggested that it would take an atom-sized PBH approximately 0.01 ms to pass through a human body. Despite that very quick route, the PBH’s gravitational effects would cause the person to shrink by several inches in the process, causing severe damage and immediate death.
Rahvar, meanwhile, points to a different but equally fatal consequence – the burning of flesh around the axis of the PBH’s path in the body. “What will kill a human is not the direct swallowing of the human body inside the primordial black hole. The main cause of death would be burning part of the body with high-energy photons released from the matter accreting into the black hole.”
With his initial study complete, Rahvar is now investigating whether it is possible to detect PBHs in the Milky Way, using space-based telescopes, by means of the microlensing effect that they would have on foreground stars. “With space-based telescopes such as the Nancy Grace Roman Space Telescope or JWST, we may detect or rule out small-mass primordial black holes in the near future,” he explains.
Rahvar is also modelling how PBHs might interact with the Oort cloud that sits at the outskirts of the solar system – and whether the tiny but massive objects might be able to fling icy material from the cloud into the heart of the solar system. Of course, if they can, one obvious question would remain – what would happen if the icy material hits Earth? For the answer to that, you’ll have to watch this space.