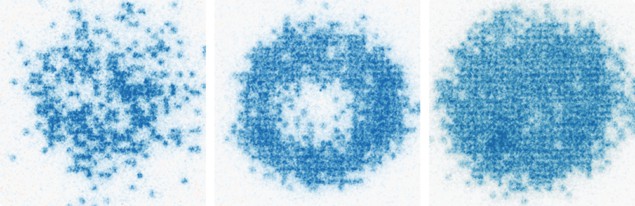
Researchers in the US have taken images of individual atoms in an ultracold fermionic gas as it makes the transition from a metallic phase to a band insulator and then to a Mott insulator. This is the first study of such a transition in a fermionic gas to be made with single-site and single-particle resolution. While such experiments are routinely done using ultracold bosonic atoms, doing the same with fermions is more challenging because they are difficult to cool. However, the rewards for physicists could be greater because fermionic atoms are a closer match to electrons in a solid, and therefore such experiments could shed light on poorly understood solid systems such as high-temperature superconductors.
Fermions are particles that have half-integer spin, and are constrained by the Pauli exclusion principle, which dictates that no two identical fermions can occupy the same quantum state simultaneously. Fermions include many elementary particles such as quarks, electrons, protons and neutrons, and so their collective behaviour is responsible for the structure of the elements in the periodic table, high-temperature superconductors, the properties of nuclear matter and much more.
Cooling off
Studying strongly interacting systems of fermionic atoms in ultracold gases should allow physicists to study a wide range of collective behaviours. However, creating such systems is difficult because the exclusion principle means that each fermion added to a system comes in at an increasingly higher energy, making such gases very difficult to cool and image. It was only last year that researchers managed to create and image an ultracold fermionic gas in an optical lattice, where single fermions were clearly resolved and certain interactions directly detected (see “Fermionic microscope sees first light“).
A fermionic microscope allows quantum physicists to delve into the intricacies of how strong interactions between fermions lead to complex quantum many-body systems such as spin liquids and d-wave superconductors. Probing such systems with single-site resolution in a lattice should offer key insights into these phenomena.
Fermionic transitions
Now, Daniel Greif, Markus Greiner and other colleagues at Harvard University in the US have created their own fermionic microscope using ultracold lithium-6 atoms that are trapped in a 2D optical lattice. They then used it to take images of the atoms as the system makes the transition from a metallic phase to a band insulator, and then to an interaction-dominated Mott-insulator phase.
When the interaction energy of the gas is small compared with the kinetic energy, the atoms are largely free to move around, although no two fermions will occupy the same lattice site unless their spins are different. But as the interaction energy between atoms is increased to cause a greater repulsion between atoms – limiting the atoms’ ability to hop between lattice sites – phase transitions occur.
In the Mott-insulator state, for example, the repulsion is so strong that an atom cannot hop into a neighbouring site that contains an atom. Because all sites contain one atom, the atoms are unable to move and behave like an insulator in an analogy to electrons in a solid. This kind of self-arranged quantum state could, in principle, have very low entropy, which makes it a good starting point to engineer other many-body quantum systems of interest. Normally, the atoms’ spins do not influence one another during a Mott phase and only their relative positions have any influence. However, at very low temperatures a phenomenon known as “superexchange” kicks in and the spins should be ordered in an alternating and anti-aligned pattern.
New view
Team member Sebastian Blatt told physicsworld.com that the team has extended a fermionic microscope technique developed last year to fermionic lithium-6. “The only two alkali metals that can be laser-cooled and have fermionic isotopes are lithium-6 and potassium-40,” says Blatt. But he also points out that the metals’ atomic structure does not lend itself to the fluorescence-imaging technique used in quantum-gas microscopes, so getting the imaging technique to work at the single-site- and single-atom-resolved level was “a major step forward”.
“The new thing about the imaging is that we can now measure local variables and correlations in this quantum many-body system,” says Blatt, explaining that the fermionic character of the atoms is important because it leads to very different states of matter than those formed in bosonic systems. “Our fermionic lithium-6 atoms are also much closer in character to electrons in solids than typically used bosonic atoms such as rubidium-87. This is both because lithium-6 is a fermion, and because of its relatively small mass,” he adds. This ability to experimentally observe local changes and transitions in ultracold fermionic gasses will help to improve our understanding of fermionic many-body systems.
The measurements are described in Science.