Flash Physics is our daily pick of the latest need-to-know developments from the global physics community selected by Physics World‘s team of editors and reporters

US university reactor to make molybdenum-99
The University of Missouri Research Reactor (MURR) has unveiled plans to make molybdenum-99. This is used to make the medical-imaging isotope technetium-99m, which could soon be in short supply in North America because of the upcoming closure of a reactor in Canada. MURR has filed an application with the US Nuclear Regulatory Commission to make the isotope using technology developed by US-based General Atomics. This will involve placing low enriched uranium targets inside the reactor and then extracting molybdenum-99 from the irradiated targets. The molybdenum-99, which has a half-life of 66 days, will then be transported 1600 km to Ottawa, where it will be further purified by Canada-based Nordion. The isotope is then distributed to radiopharmaceutical manufacturers, who integrate the molybdenum into technetium-99m generators, which are shipped to hospitals. The plan is a response to possible shortages of molybdenum-99 that could occur once the NRU reactor in Chalk River, Canada, shuts down in March 2018. NRU currently makes most of the molybdenum-99 used in North America and officials at MURR say their proposal could supply nearly half of the US demand for the isotope.
Shining a light on radiotherapy side effects
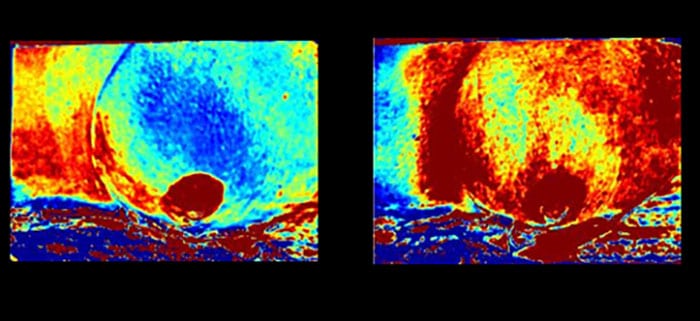
An LED-based device allows scientists to monitor skin damage caused by radiotherapy during breast-cancer treatment. Patients often undergo radiation therapy after surgery or chemotherapy to kill any remaining cancerous cells. Unfortunately, the treatment can cause significant skin afflictions including irritation, peeling, blistering, permanent discolouration and tissue thickening. There are currently no methods to predict the severity of reactions, so researchers at the University of California, Irvine (UC Irvine) in the US have begun using Spatial Frequency Domain Imaging (SFDI) devices to monitor and characterize the damage. SFDI uses the fact that light absorbs and scatters to varying degrees depending upon the properties of the target object. Detecting the reflected light gives information about those properties. In the current work, Anaïs Leproux and colleagues are using an SFDI device, originally developed by David Cuccia, formally of UC Irvine and founder of Modulated Imaging. To monitor breast tissues, the team use low-power, LED light of eight visible to near-infrared wavelengths. By shining the light in certain patterns using a digital micro-mirror, a camera can detect varying reflectance more accurately. “Since we use several wavelengths of light, we perform spectroscopy and obtain the content of melanin, tissue hemoglobin, in the de-oxygenated and oxygenated state, from which we can calculate the total blood volume and oxygen saturation in the tissue,” says Leproux. The non-invasive technique measures 3–5 mm into the skin. The team addressed concerns about exposing skin to additional radiation by calculating that 10 measurements is the equivalent of two seconds in the sun. They hope that by characterizing and monitoring the skin damage, they can gain a better understanding of the processes involved and potentially predict patients’ reactions. The work is being presented at next week’s OSA Biophotonics Congress: Optics in the Life Sciences meeting in San Diego, California.
Entangled photons created in two different places
Photons created in entangled pairs in a nonlinear crystal can emerge at two different points in space, say physicists in the UK who have studied a special case of a process called spontaneous parametric down-conversion (SPDC). Their finding contradicts a general assumption that such photons are created at the same place in the crystal. SPDC involves firing higher-energy photons into a special type of crystal, which results in the production of two lower-energy photons. This is a quantum-mechanical process and the two photons emerge in an entangled state, which means that as they fly off in different directions they maintain a relationship that is stronger than that allowed by classical physics. Such entangled pairs can then be used in a number of applications including quantum computing and quantum cryptography. Kayn Forbes, Jack Ford and David Andrews of the University of East Anglia looked at a specific type of SPDC called degenerate down-conversion in which the two electrons have the same energy. “Until now, it has been assumed that such paired photons come from the same location,” says Andrews. The trio identified a process involving the propagation of virtual photons in the crystal that results in photons being emitted from two different places. Andrews describes this as “a new positional uncertainty of a fundamental quantum origin,” adding: “Everything has a certain quantum ‘fuzziness’ to it, and photons are not the hard little bullets of light that are popularly imagined.” The study will be described in Physical Review Letters.
- You can find all our daily Flash Physics posts in the website’s news section, as well as on Twitter and Facebook using #FlashPhysics.