
Inspired by microstructures on a gecko’s toepad, engineers in the US have created a directional adhesive with a grip that is 100 times larger in one direction than it is in the opposite direction. Based at Stanford University, the researchers say that their work shows that bio-inspired spatial variation can provide artificial microstructures with advanced properties and help close the performance gap between synthetic and natural materials.
Geckos are renowned for their ability to climb up smooth vertical surfaces like glass using microscopic hair-like setae that cover their toes. These setae branch, becoming finer and finer, before eventually terminating in flat spatulae that get so close to the surface that they create a dry adhesive that sticks through van der Waals forces. But, geckos are also able to easily and rapidly detach their feet as they scale obstacles.
In 2006, researchers at Lewis & Clark College and Stanford University, discovered that a gecko’s setae only grip in one direction. The setae all curve in the same direction and when the branched ends are pushed against a surface they spread out and grip. But if the setae are turned around, because of the curvature, none of the spatulae make contact with the surface and the hair just slides. This directional adhesion means that the toes grips tightly when loaded, but as it runs, a gecko can easily take the weight off a foot and slide the toes forwards.
One-way friction
Inspired by this work, Stanford’s Arul Suresh and colleagues have created an adhesive with similar one-way friction by varying its surface microstructure so that its shape changes when pulled in different directions.
Using a silicone elastomer, they sculpted a material that consists of a series of microscale wedges. When pulled along a surface in one direction, the wedges are drawn towards the surface, creating close contact and friction. But, a few of the wedges are larger and longer than the others. When the adhesive is pulled in the opposite direction these features curl over the other wedges and push them away from the surface, preventing close contact and drastically reducing friction.
Tests showed that the material has a 100:1 friction ratio: a 24 x 25 mm patch was able to hold a 2.3 kg weight in one direction, but only 22 g in the reverse direction.
Shuffling robotic inchworm
To demonstrate the practical use of the material, Suresh told Physics World that the team built a robot that moves like an inchworm – a caterpillar that moves forward by arching and straightening its body. “Normally, for a robot like this to work, it needs to be complicated enough to pick up each foot and move it forward, or it will waste a lot of energy by sliding each foot forward against friction,” he explains. “By placing a material with one-way friction on the feet, a robot like this can still push with large forces but can easily slide the feet forward when repositioning using a simple linkage. This allows a robot to “shuffle” while still pulling loads, instead of needing to pick up the feet and take steps.”
Suresh says that a one-way friction material could also be used to turn any surface into a ratchet. “This would allow robots, or other devices, to move easily in the low-friction direction, but also resist strong forces pushing them back,” he explains.
Looking at nearly any natural system, what’s immediately striking is how complicated everything is – at least, compared to the products we typically use every day
Arul Suresh
Natural materials are often very complex, with surface microstructures that show a lot of spatial variation. Iridescence, hydrophobicity and anti-drag properties, for example, all occur in biological structures through microscopic surface variations. In artificial systems, however, surface microstructures tend to be uniform – in part because manufacturing processes favour simplicity.
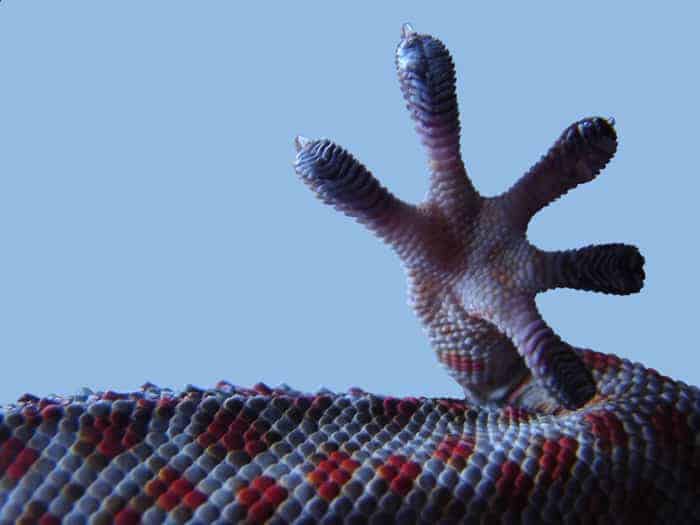
Static electricity helps geckos get a grip
According to the team, their work shows how spatially variation can provide artificial microstructures with advanced properties. They add that researchers should look to biological systems to inspire them to create the next generation of microstructures.
“Looking at nearly any natural system, what’s immediately striking is how complicated everything is – at least, compared to the products we typically use every day,” says Suresh. “Nature has the ability to tailor solutions cell-by-cell to meet particular challenges. Research in other biological systems has shown that the small variations do affect the capabilities of those systems.”
The research is described in Journal of the Royal Society Interface.